Switch to List View
Image and Video Gallery
This is a searchable collection of scientific photos, illustrations, and videos. The images and videos in this gallery are licensed under Creative Commons Attribution Non-Commercial ShareAlike 3.0. This license lets you remix, tweak, and build upon this work non-commercially, as long as you credit and license your new creations under identical terms.
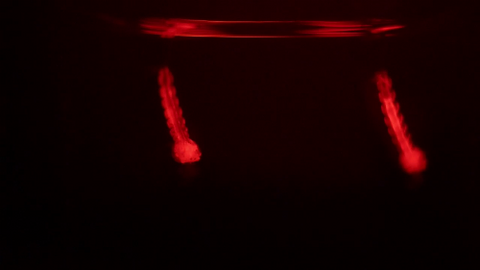
6771: Culex quinquefasciatus mosquito larvae
6771: Culex quinquefasciatus mosquito larvae
Mosquito larvae with genes edited by CRISPR swimming in water. This species of mosquito, Culex quinquefasciatus, can transmit West Nile virus, Japanese encephalitis virus, and avian malaria, among other diseases. The researchers who took this video optimized the gene-editing tool CRISPR for Culex quinquefasciatus that could ultimately help stop the mosquitoes from spreading pathogens. The work is described in the Nature Communications paper "Optimized CRISPR tools and site-directed transgenesis towards gene drive development in Culex quinquefasciatus mosquitoes" by Feng et al. Related to images 6769 and 6770.
Valentino Gantz, University of California, San Diego.
View Media
2433: Fruit fly sperm cells
2433: Fruit fly sperm cells
Developing fruit fly spermatids require caspase activity (green) for the elimination of unwanted organelles and cytoplasm via apoptosis.
Hermann Steller, Rockefeller University
View Media
5760: Annotated TEM cross-section of C. elegans (roundworm)
5760: Annotated TEM cross-section of C. elegans (roundworm)
The worm Caenorhabditis elegans is a popular laboratory animal because its small size and fairly simple body make it easy to study. Scientists use this small worm to answer many research questions in developmental biology, neurobiology, and genetics. This image, which was taken with transmission electron microscopy (TEM), shows a cross-section through C. elegans, revealing various internal structures labeled in the image. You can find a high-resolution image without the annotations at image 5759.
The image is from a figure in an article published in the journal eLife.
The image is from a figure in an article published in the journal eLife.
Piali Sengupta, Brandeis University
View Media
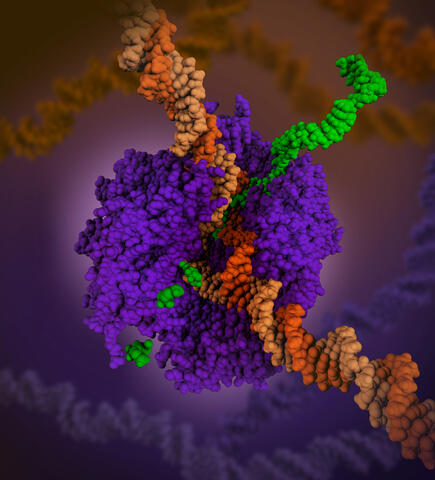
6993: RNA polymerase
6993: RNA polymerase
RNA polymerase (purple) is a complex enzyme at the heart of transcription. During this process, the enzyme unwinds the DNA double helix and uses one strand (darker orange) as a template to create the single-stranded messenger RNA (green), later used by ribosomes for protein synthesis.
From the RNA polymerase II elongation complex of Saccharomyces cerevisiae (PDB entry 1I6H) as seen in PDB-101's What is a Protein? video.
From the RNA polymerase II elongation complex of Saccharomyces cerevisiae (PDB entry 1I6H) as seen in PDB-101's What is a Protein? video.
Amy Wu and Christine Zardecki, RCSB Protein Data Bank.
View Media
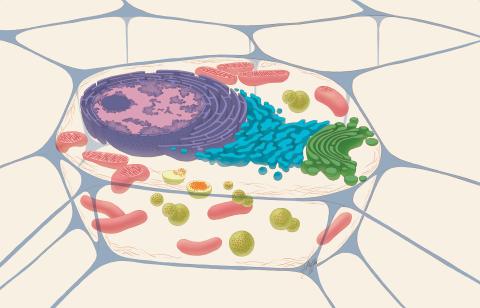
1274: Animal cell
1274: Animal cell
A typical animal cell, sliced open to reveal a cross-section of organelles.
Judith Stoffer
View Media
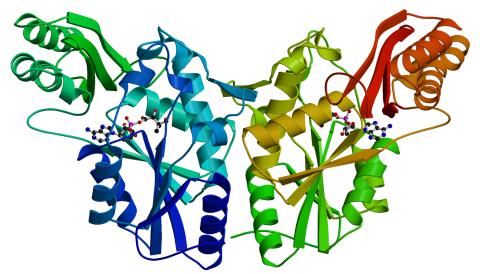
2383: PanC from M. tuberculosis
2383: PanC from M. tuberculosis
Model of an enzyme, PanC, that is involved in the last step of vitamin B5 biosynthesis in Mycobacterium tuberculosis. PanC is essential for the growth of M. tuberculosis, which causes most cases of tuberculosis, and is therefore a potential drug target.
Mycobacterium Tuberculosis Center, PSI
View Media
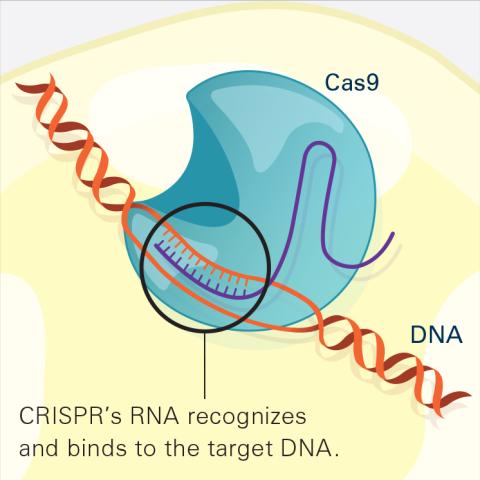
6486: CRISPR Illustration Frame 2
6486: CRISPR Illustration Frame 2
This illustration shows, in simplified terms, how the CRISPR-Cas9 system can be used as a gene-editing tool. The CRISPR system has two components joined together: a finely tuned targeting device (a small strand of RNA programmed to look for a specific DNA sequence) and a strong cutting device (an enzyme called Cas9 that can cut through a double strand of DNA). In this frame (2 of 4), the CRISPR machine locates the target DNA sequence once inserted into a cell.
For an explanation and overview of the CRISPR-Cas9 system, see the iBiology video, and find the full CRIPSR illustration here.
For an explanation and overview of the CRISPR-Cas9 system, see the iBiology video, and find the full CRIPSR illustration here.
National Institute of General Medical Sciences.
View Media
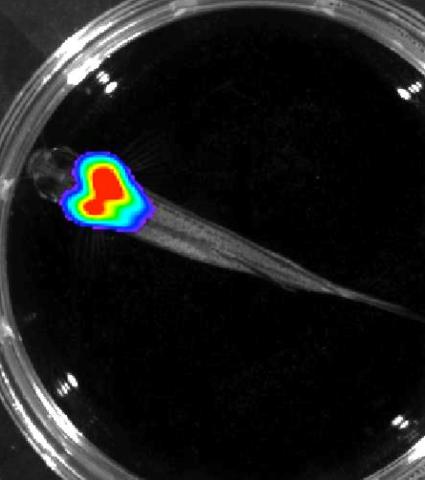
3557: Bioluminescent imaging in adult zebrafish - overhead view
3557: Bioluminescent imaging in adult zebrafish - overhead view
Luciferase-based imaging enables visualization and quantification of internal organs and transplanted cells in live adult zebrafish. In this image, a cardiac muscle-restricted promoter drives firefly luciferase expression.
For imagery of both the lateral and overhead view go to 3556.
For imagery of the lateral view go to 3558.
For more information about the illumated area go to 3559.
For imagery of both the lateral and overhead view go to 3556.
For imagery of the lateral view go to 3558.
For more information about the illumated area go to 3559.
Kenneth Poss, Duke University
View Media
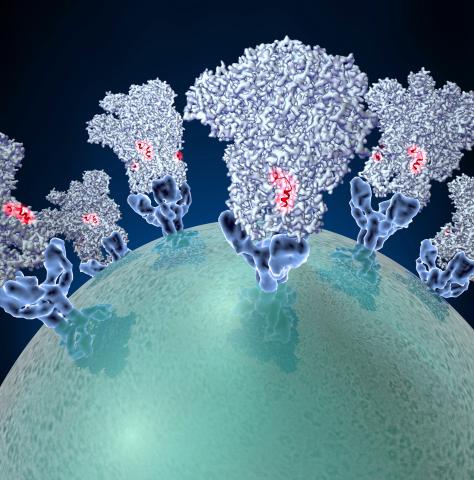
3753: Coronavirus spike protein structure
3753: Coronavirus spike protein structure
Coronaviruses are enveloped viruses responsible for 30 percent of mild respiratory infections and atypical deadly pneumonia in humans worldwide. These deadly pneumonia include those caused by infections with severe acute respiratory syndrome coronavirus (SARS-CoV) and Middle East respiratory syndrome coronavirus (MERS-CoV). The coronavirus spike glycoprotein mediates virus entry into cells and represents an important therapeutic target. The illustration shows a viral membrane decorated with spike glycoproteins; highlighted in red is a potential neutralization site, which is a protein sequence that might be used as a target for vaccines to combat viruses such as MERS-CoV and other coronaviruses.
Melody Campbell, UCSF
View Media
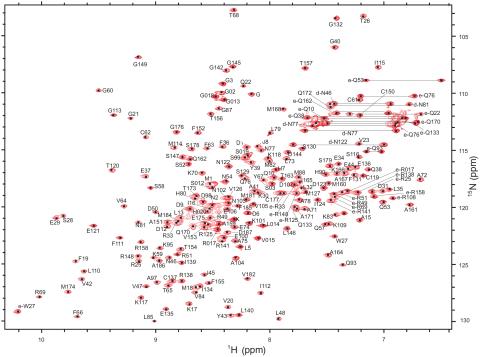
2299: 2-D NMR
2299: 2-D NMR
A two-dimensional NMR spectrum of a protein, in this case a 2D 1H-15N HSQC NMR spectrum of a 228 amino acid DNA/RNA-binding protein.
Dr. Xiaolian Gao's laboratory at the University of Houston
View Media
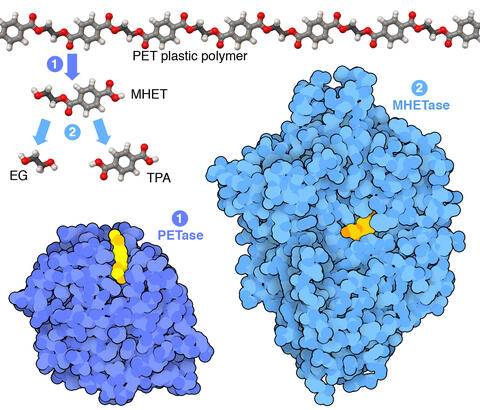
7000: Plastic-eating enzymes
7000: Plastic-eating enzymes
PETase enzyme degrades polyester plastic (polyethylene terephthalate, or PET) into monohydroxyethyl terephthalate (MHET). Then, MHETase enzyme degrades MHET into its constituents ethylene glycol (EG) and terephthalic acid (TPA).
Find these in the RCSB Protein Data Bank: PET hydrolase (PDB entry 5XH3) and MHETase (PDB entry 6QGA).
Find these in the RCSB Protein Data Bank: PET hydrolase (PDB entry 5XH3) and MHETase (PDB entry 6QGA).
Amy Wu and Christine Zardecki, RCSB Protein Data Bank.
View Media
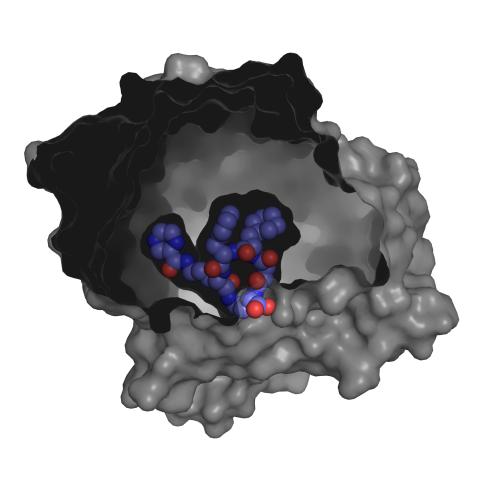
3415: X-ray co-crystal structure of Src kinase bound to a DNA-templated macrocycle inhibitor 3
3415: X-ray co-crystal structure of Src kinase bound to a DNA-templated macrocycle inhibitor 3
X-ray co-crystal structure of Src kinase bound to a DNA-templated macrocycle inhibitor. Related to 3413, 3414, 3416, 3417, 3418, and 3419.
Markus A. Seeliger, Stony Brook University Medical School and David R. Liu, Harvard University
View Media
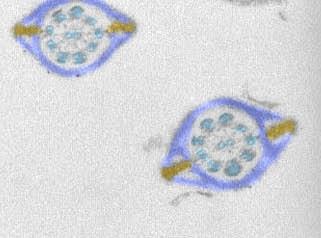
1191: Mouse sperm sections
1191: Mouse sperm sections
This transmission electron micrograph shows sections of mouse sperm tails, or flagella.
Tina Weatherby Carvalho, University of Hawaii at Manoa
View Media
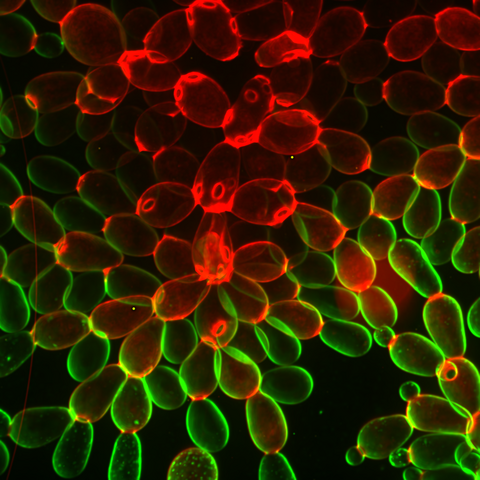
6969: Snowflake yeast 1
6969: Snowflake yeast 1
Multicellular yeast called snowflake yeast that researchers created through many generations of directed evolution from unicellular yeast. Stained cell membranes (green) and cell walls (red) reveal the connections between cells. Younger cells take up more cell membrane stain, while older cells take up more cell wall stain, leading to the color differences seen here. This image was captured using spinning disk confocal microscopy.
Related to images 6970 and 6971.
Related to images 6970 and 6971.
William Ratcliff, Georgia Institute of Technology.
View Media
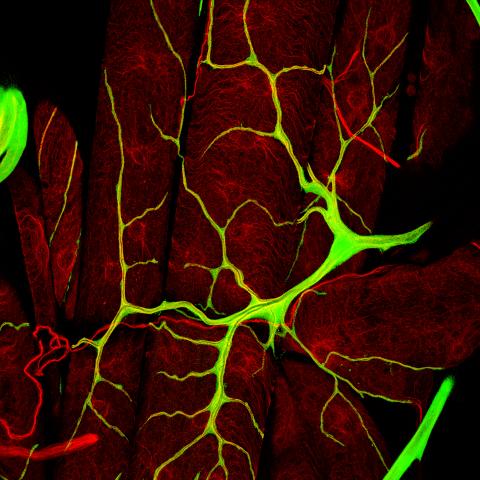
3615: An insect tracheal cell delivers air to muscles
3615: An insect tracheal cell delivers air to muscles
Insects like the fruit fly use an elaborate network of branching tubes called trachea (green) to transport oxygen throughout their bodies. Fruit flies have been used in biomedical research for more than 100 years and remain one of the most frequently studied model organisms. They have a large percentage of genes in common with us, including hundreds of genes that are associated with human diseases.
This image was part of the Life: Magnified exhibit that ran from June 3, 2014, to January 21, 2015, at Dulles International Airport.
This image was part of the Life: Magnified exhibit that ran from June 3, 2014, to January 21, 2015, at Dulles International Airport.
Jayan Nair and Maria Leptin, European Molecular Biology Laboratory, Heidelberg, Germany
View Media
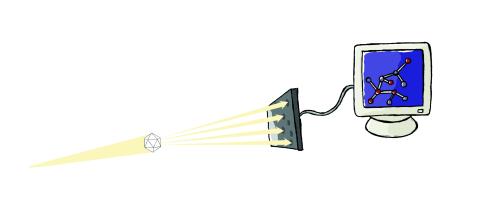
2511: X-ray crystallography
2511: X-ray crystallography
X-ray crystallography allows researchers to see structures too small to be seen by even the most powerful microscopes. To visualize the arrangement of atoms within molecules, researchers can use the diffraction patterns obtained by passing X-ray beams through crystals of the molecule. This is a common way for solving the structures of proteins. See image 2512 for a labeled version of this illustration. Featured in The Structures of Life.
Crabtree + Company
View Media
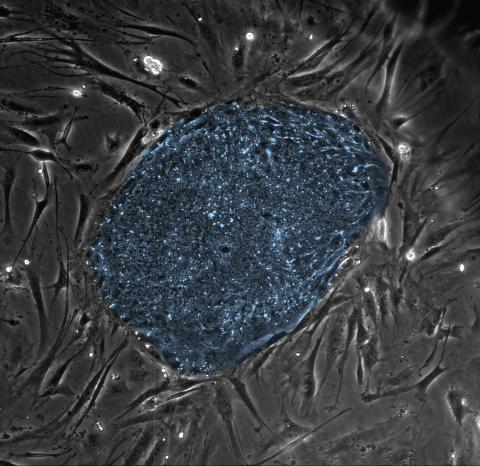
2608: Human embryonic stem cells
2608: Human embryonic stem cells
The center cluster of cells, colored blue, shows a colony of human embryonic stem cells. These cells, which arise at the earliest stages of development, are capable of differentiating into any of the 220 types of cells in the human body and can provide access to cells for basic research and potential therapies. This image is from the lab of the University of Wisconsin-Madison's James Thomson.
James Thomson, University of Wisconsin-Madison
View Media
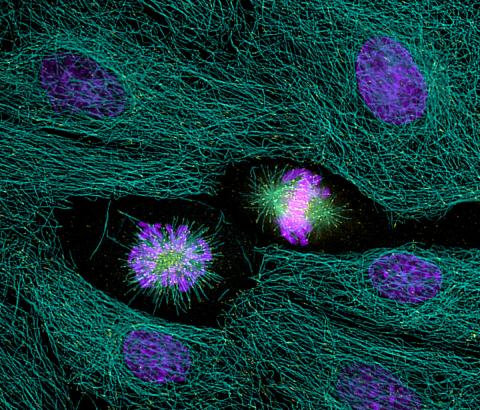
2429: Highlighted cells
2429: Highlighted cells
The cytoskeleton (green) and DNA (purple) are highlighed in these cells by immunofluorescence.
Torsten Wittmann, Scripps Research Institute
View Media
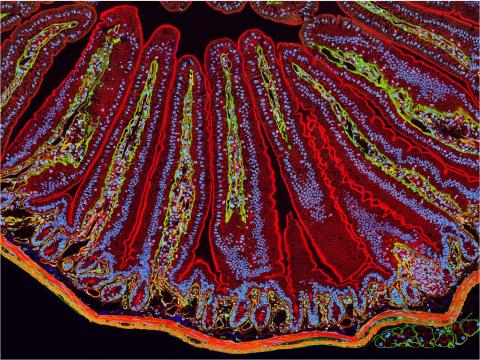
3389: NCMIR Intestine-1
3389: NCMIR Intestine-1
The small intestine is where most of our nutrients from the food we eat are absorbed into the bloodstream. The walls of the intestine contain small finger-like projections called villi which increase the organ's surface area, enhancing nutrient absorption. It consists of the duodenum, which connects to the stomach, the jejenum and the ileum, which connects with the large intestine. Related to image 3390.
Tom Deerinck, National Center for Microscopy and Imaging Research (NCMIR)
View Media
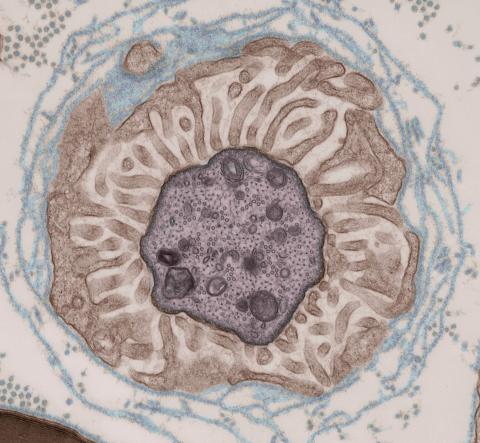
3740: Transmission electron microscopy showing cross-section of the node of Ranvier
3740: Transmission electron microscopy showing cross-section of the node of Ranvier
Nodes of Ranvier are short gaps in the myelin sheath surrounding myelinated nerve cells (axons). Myelin insulates axons, and the node of Ranvier is where the axon is exposed to the extracellular environment, allowing for the transmission of action potentials at these nodes via ion flows between the inside and outside of the axon. The image shows a cross-section through the node, with the surrounding extracellular matrix encasing and supporting the axon shown in cyan.
Tom Deerinck, National Center for Microscopy and Imaging Research (NCMIR)
View Media
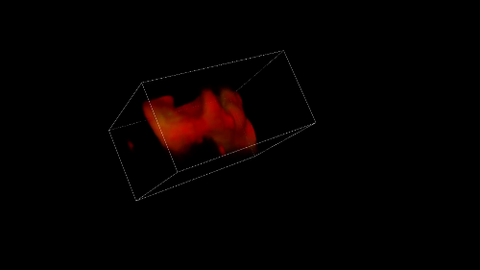
5877: Misfolded proteins in mitochondria, 3-D video
5877: Misfolded proteins in mitochondria, 3-D video
Three-dimensional image of misfolded proteins (green) within mitochondria (red). Related to image 5878. Learn more in this press release by The American Association for the Advancement of Science.
Rong Li, Department of Chemical and Biomolecular Engineering, Whiting School of Engineering, Johns Hopkins University
View Media
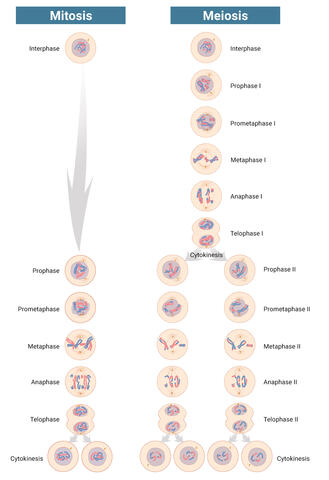
6788: Mitosis and meiosis compared-labeled
6788: Mitosis and meiosis compared-labeled
Meiosis is used to make sperm and egg cells. During meiosis, a cell's chromosomes are copied once, but the cell divides twice. During mitosis, the chromosomes are copied once, and the cell divides once. For simplicity, cells are illustrated with only three pairs of chromosomes.
See image 1333 for an unlabeled version of this illustration.
See image 1333 for an unlabeled version of this illustration.
Judith Stoffer
View Media
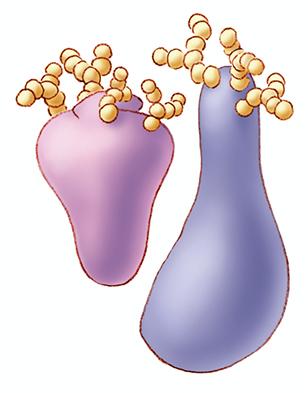
1270: Glycoproteins
1270: Glycoproteins
About half of all human proteins include chains of sugar molecules that are critical for the proteins to function properly. Appears in the NIGMS booklet Inside the Cell.
Judith Stoffer
View Media
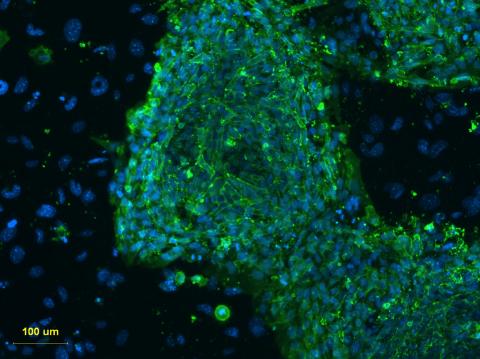
3274: Human embryonic stem cells on feeder cells
3274: Human embryonic stem cells on feeder cells
This fluorescent microscope image shows human embryonic stem cells whose nuclei are stained green. Blue staining shows the surrounding supportive feeder cells. Image and caption information courtesy of the California Institute for Regenerative Medicine. See related image 3275.
Michael Longaker lab, Stanford University School of Medicine, via CIRM
View Media
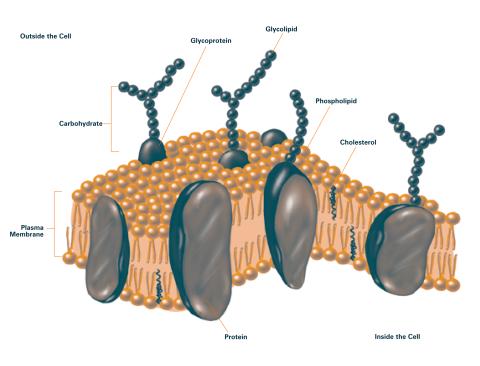
2524: Plasma membrane (with labels)
2524: Plasma membrane (with labels)
The plasma membrane is a cell's protective barrier. See image 2523 for an unlabeled version of this illustration. Featured in The Chemistry of Health.
Crabtree + Company
View Media
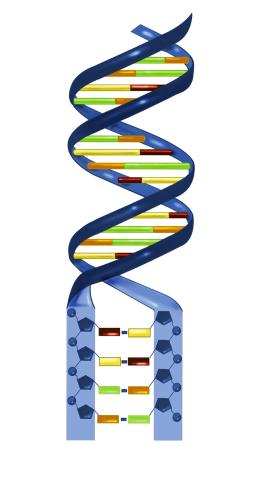
2541: Nucleotides make up DNA
2541: Nucleotides make up DNA
DNA consists of two long, twisted chains made up of nucleotides. Each nucleotide contains one base, one phosphate molecule, and the sugar molecule deoxyribose. The bases in DNA nucleotides are adenine, thymine, cytosine, and guanine. See image 2542 for a labeled version of this illustration. Featured in The New Genetics.
Crabtree + Company
View Media
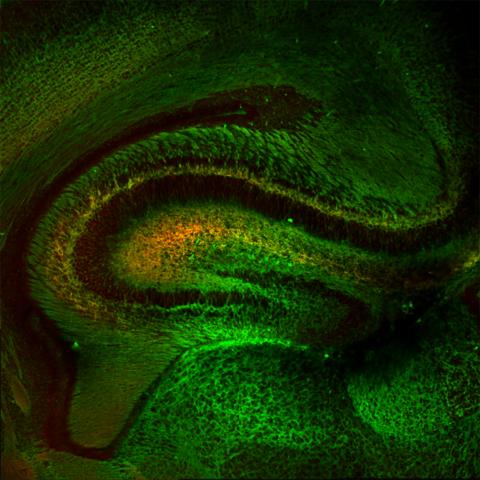
5886: Mouse Brain Cross Section
5886: Mouse Brain Cross Section
The brain sections are treated with fluorescent antibodies specific to a particular protein and visualized using serial electron microscopy (SEM).
Anton Maximov, The Scripps Research Institute, La Jolla, CA
View Media
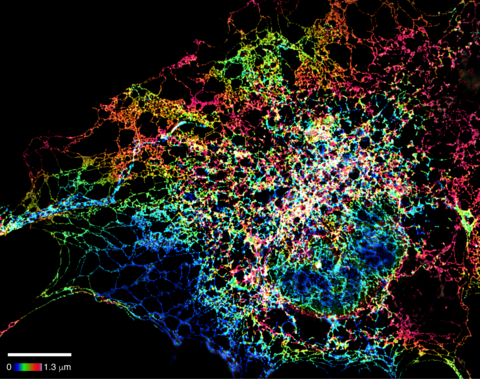
5855: Dense tubular matrices in the peripheral endoplasmic reticulum (ER) 1
5855: Dense tubular matrices in the peripheral endoplasmic reticulum (ER) 1
Superresolution microscopy work on endoplasmic reticulum (ER) in the peripheral areas of the cell showing details of the structure and arrangement in a complex web of tubes. The ER is a continuous membrane that extends like a net from the envelope of the nucleus outward to the cell membrane. The ER plays several roles within the cell, such as in protein and lipid synthesis and transport of materials between organelles. The ER has a flexible structure to allow it to accomplish these tasks by changing shape as conditions in the cell change. Shown here an image created by super-resolution microscopy of the ER in the peripheral areas of the cell showing details of the structure and the arrangements in a complex web of tubes. Related to images 5856 and 5857.
Jennifer Lippincott-Schwartz, Howard Hughes Medical Institute Janelia Research Campus, Virginia
View Media
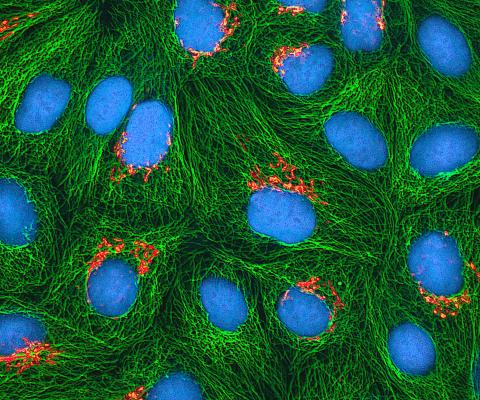
3522: HeLa cells
3522: HeLa cells
Multiphoton fluorescence image of cultured HeLa cells with a fluorescent protein targeted to the Golgi apparatus (orange), microtubules (green) and counterstained for DNA (cyan). Nikon RTS2000MP custom laser scanning microscope. See related images 3518, 3519, 3520, 3521.
National Center for Microscopy and Imaging Research (NCMIR)
View Media
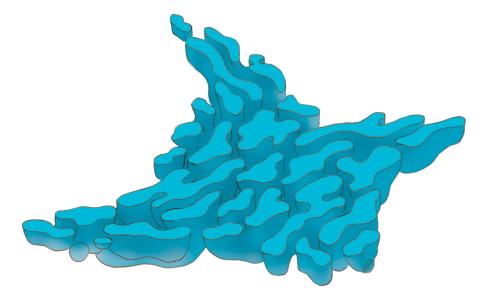
1292: Smooth ER
1292: Smooth ER
The endoplasmic reticulum comes in two types: Rough ER is covered with ribosomes and prepares newly made proteins; smooth ER specializes in making lipids and breaking down toxic molecules.
Judith Stoffer
View Media
1084: Natcher Building 04
1084: Natcher Building 04
NIGMS staff are located in the Natcher Building on the NIH campus.
Alisa Machalek, National Institute of General Medical Sciences
View Media
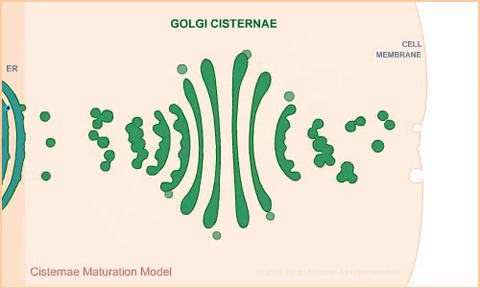
1307: Cisternae maturation model
1307: Cisternae maturation model
Animation for the cisternae maturation model of Golgi transport.
Judith Stoffer
View Media
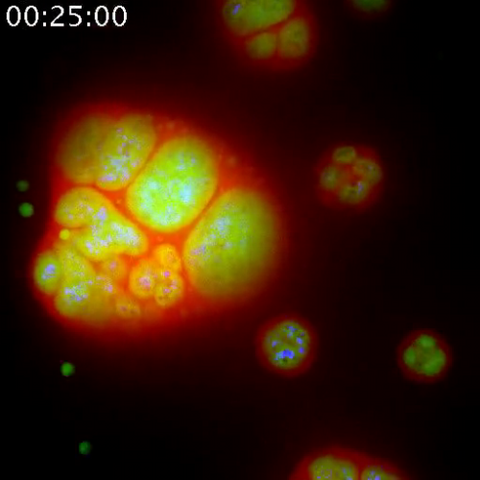
3789: Nucleolus subcompartments spontaneously self-assemble 1
3789: Nucleolus subcompartments spontaneously self-assemble 1
The nucleolus is a small but very important protein complex located in the cell's nucleus. It forms on the chromosomes at the location where the genes for the RNAs are that make up the structure of the ribosome, the indispensable cellular machine that makes proteins from messenger RNAs.
However, how the nucleolus grows and maintains its structure has puzzled scientists for some time. It turns out that even though it looks like a simple liquid blob, it's rather well-organized, consisting of three distinct layers: the fibrillar center, where the RNA polymerase is active; the dense fibrillar component, which is enriched in the protein fibrillarin; and the granular component, which contains a protein called nucleophosmin. Researchers have now discovered that this multilayer structure of the nucleolus arises from difference in how the proteins in each compartment mix with water and with each other. These differences let them readily separate from each other into the three nucleolus compartments.
This video of nucleoli in the eggs of a commonly used lab animal, the frog Xenopus laevis, shows how each of the compartments (the granular component is shown in red, the fibrillarin in yellow-green, and the fibrillar center in blue) spontaneously fuse with each other on encounter without mixing with the other compartments. For more details on this research, see this press release from Princeton. Related to video 3791, image 3792 and image 3793.
However, how the nucleolus grows and maintains its structure has puzzled scientists for some time. It turns out that even though it looks like a simple liquid blob, it's rather well-organized, consisting of three distinct layers: the fibrillar center, where the RNA polymerase is active; the dense fibrillar component, which is enriched in the protein fibrillarin; and the granular component, which contains a protein called nucleophosmin. Researchers have now discovered that this multilayer structure of the nucleolus arises from difference in how the proteins in each compartment mix with water and with each other. These differences let them readily separate from each other into the three nucleolus compartments.
This video of nucleoli in the eggs of a commonly used lab animal, the frog Xenopus laevis, shows how each of the compartments (the granular component is shown in red, the fibrillarin in yellow-green, and the fibrillar center in blue) spontaneously fuse with each other on encounter without mixing with the other compartments. For more details on this research, see this press release from Princeton. Related to video 3791, image 3792 and image 3793.
Nilesh Vaidya, Princeton University
View Media
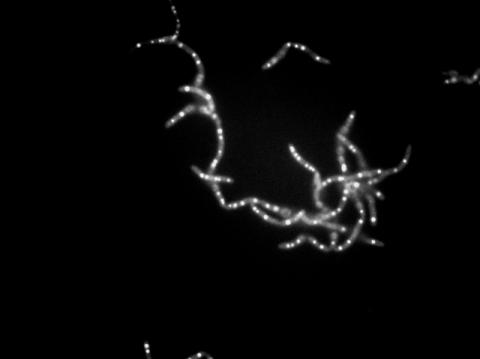
3481: Bacillus anthracis being killed
3481: Bacillus anthracis being killed
Bacillus anthracis (anthrax) cells being killed by a fluorescent trans-translation inhibitor, which disrupts bacterial protein synthesis. The inhibitor is naturally fluorescent and looks blue when it is excited by ultraviolet light in the microscope. This is a black-and-white version of Image 3525.
John Alumasa, Keiler Laboratory, Pennsylvania State University
View Media
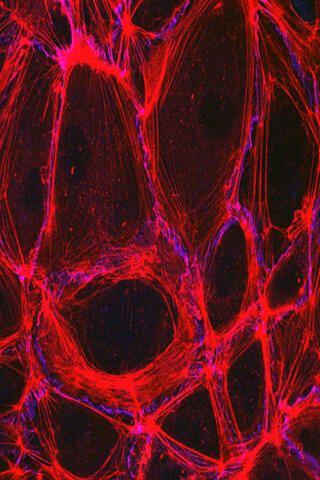
3633: Cells lining the blood vessel walls
3633: Cells lining the blood vessel walls
The structure of the endothelium, the thin layer of cells that line our arteries and veins, is visible here. The endothelium is like a gatekeeper, controlling the movement of materials into and out of the bloodstream. Endothelial cells are held tightly together by specialized proteins that function like strong ropes (red) and others that act like cement (blue).
This image was part of the Life: Magnified exhibit that ran from June 3, 2014, to January 21, 2015, at Dulles International Airport.
This image was part of the Life: Magnified exhibit that ran from June 3, 2014, to January 21, 2015, at Dulles International Airport.
Christopher V. Carman and Roberta Martinelli, Harvard Medical School.
View Media
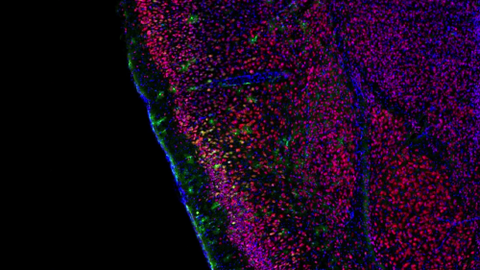
6781: Video of Calling Cards in a mouse brain
6781: Video of Calling Cards in a mouse brain
The green spots in this mouse brain are cells labeled with Calling Cards, a technology that records molecular events in brain cells as they mature. Understanding these processes during healthy development can guide further research into what goes wrong in cases of neuropsychiatric disorders. Also fluorescently labeled in this video are neurons (red) and nuclei (blue). Calling Cards and its application are described in the Cell paper “Self-Reporting Transposons Enable Simultaneous Readout of Gene Expression and Transcription Factor Binding in Single Cells” by Moudgil et al.; and the Proceedings of the National Academy of Sciences paper “A viral toolkit for recording transcription factor–DNA interactions in live mouse tissues” by Cammack et al. This video was created for the NIH Director’s Blog post The Amazing Brain: Tracking Molecular Events with Calling Cards.
Related to image
Related to image
<em>NIH Director’s Blog</em>.
View Media
2791: Anti-tumor drug ecteinascidin 743 (ET-743) with hydrogens 02
2791: Anti-tumor drug ecteinascidin 743 (ET-743) with hydrogens 02
Ecteinascidin 743 (ET-743, brand name Yondelis), was discovered and isolated from a sea squirt, Ecteinascidia turbinata, by NIGMS grantee Kenneth Rinehart at the University of Illinois. It was synthesized by NIGMS grantees E.J. Corey and later by Samuel Danishefsky. Multiple versions of this structure are available as entries 2790-2797.
Timothy Jamison, Massachusetts Institute of Technology
View Media
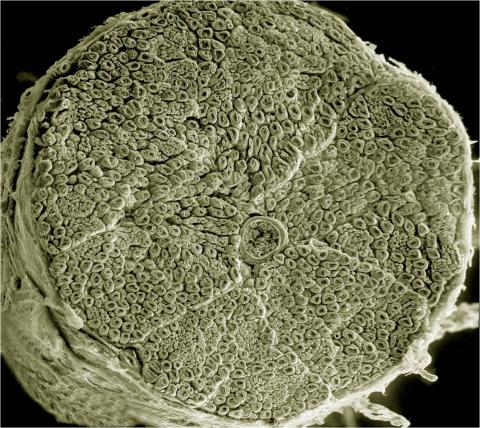
3387: NCMIR human spinal nerve
3387: NCMIR human spinal nerve
Spinal nerves are part of the peripheral nervous system. They run within the spinal column to carry nerve signals to and from all parts of the body. The spinal nerves enable all the movements we do, from turning our heads to wiggling our toes, control the movements of our internal organs, such as the colon and the bladder, as well as allow us to feel touch and the location of our limbs.
Tom Deerinck, National Center for Microscopy and Imaging Research (NCMIR)
View Media
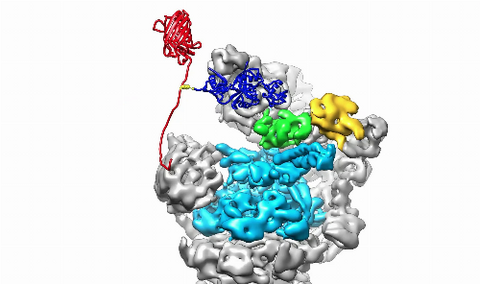
3764: Movie of the 19S proteasome subunit processing a protein substrate
3764: Movie of the 19S proteasome subunit processing a protein substrate
The proteasome is a critical multiprotein complex in the cell that breaks down and recycles proteins that have become damaged or are no longer needed. This movie shows how a protein substrate (red) is bound through its ubiquitin chain (blue) to one of the ubiquitin receptors of the proteasome (Rpn10, yellow). The substrate's flexible engagement region then gets engaged by the AAA+ motor of the proteasome (cyan), which initiates mechanical pulling, unfolding and movement of the protein into the proteasome's interior for cleavage into shorter protein pieces called peptides. During movement of the substrate, its ubiquitin modification gets cleaved off by the deubiquitinase Rpn11 (green), which sits directly above the entrance to the AAA+ motor pore and acts as a gatekeeper to ensure efficient ubiquitin removal, a prerequisite for fast protein breakdown by the 26S proteasome. Related to image 3763.
Andreas Martin, HHMI
View Media
1083: Natcher Building 03
1083: Natcher Building 03
NIGMS staff are located in the Natcher Building on the NIH campus.
Alisa Machalek, National Institute of General Medical Sciences
View Media
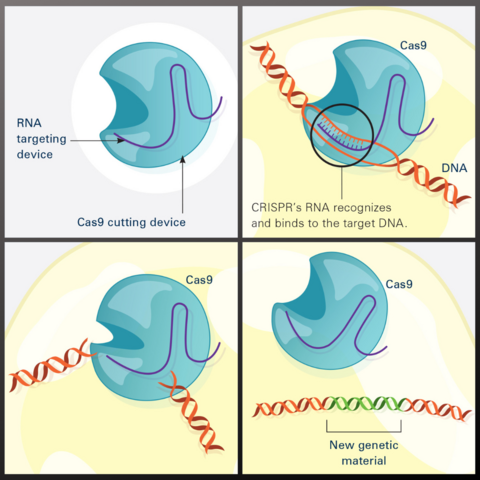
7036: CRISPR Illustration
7036: CRISPR Illustration
This illustration shows, in simplified terms, how the CRISPR-Cas9 system can be used as a gene-editing tool.
Frame 1 shows the two components of the CRISPR system: a strong cutting device (an enzyme called Cas9 that can cut through a double strand of DNA), and a finely tuned targeting device (a small strand of RNA programmed to look for a specific DNA sequence).
In frame 2, the CRISPR machine locates the target DNA sequence once inserted into a cell.
In frame 3, the Cas9 enzyme cuts both strands of the DNA.
Frame 4 shows a repaired DNA strand with new genetic material that researchers can introduce, which the cell automatically incorporates into the gap when it repairs the broken DNA.
For an explanation and overview of the CRISPR-Cas9 system, see the iBiology video.
Download the individual frames: Frame 1, Frame 2, Frame 3, and Frame 4.
Frame 1 shows the two components of the CRISPR system: a strong cutting device (an enzyme called Cas9 that can cut through a double strand of DNA), and a finely tuned targeting device (a small strand of RNA programmed to look for a specific DNA sequence).
In frame 2, the CRISPR machine locates the target DNA sequence once inserted into a cell.
In frame 3, the Cas9 enzyme cuts both strands of the DNA.
Frame 4 shows a repaired DNA strand with new genetic material that researchers can introduce, which the cell automatically incorporates into the gap when it repairs the broken DNA.
For an explanation and overview of the CRISPR-Cas9 system, see the iBiology video.
Download the individual frames: Frame 1, Frame 2, Frame 3, and Frame 4.
National Institute of General Medical Sciences.
View Media
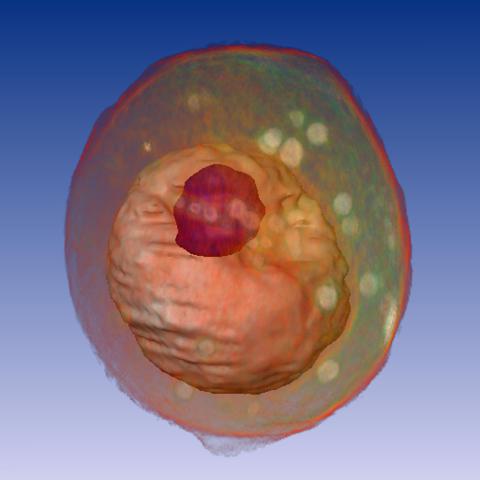
1092: Yeast cell
1092: Yeast cell
A whole yeast (Saccharomyces cerevisiae) cell viewed by X-ray microscopy. Inside, the nucleus and a large vacuole (red) are visible.
Carolyn Larabell, University of California, San Francisco and the Lawrence Berkeley National Laboratory
View Media
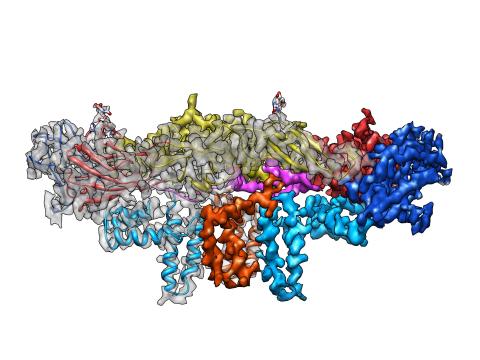
3758: Dengue virus membrane protein structure
3758: Dengue virus membrane protein structure
Dengue virus is a mosquito-borne illness that infects millions of people in the tropics and subtropics each year. Like many viruses, dengue is enclosed by a protective membrane. The proteins that span this membrane play an important role in the life cycle of the virus. Scientists used cryo-EM to determine the structure of a dengue virus at a 3.5-angstrom resolution to reveal how the membrane proteins undergo major structural changes as the virus matures and infects a host. The image shows a side view of the structure of a protein composed of two smaller proteins, called E and M. Each E and M contributes two molecules to the overall protein structure (called a heterotetramer), which is important for assembling and holding together the viral membrane, i.e., the shell that surrounds the genetic material of the dengue virus. The dengue protein's structure has revealed some portions in the protein that might be good targets for developing medications that could be used to combat dengue virus infections. For more on cryo-EM see the blog post Cryo-Electron Microscopy Reveals Molecules in Ever Greater Detail. You can watch a rotating view of the dengue virus surface structure in video 3748.
Hong Zhou, UCLA
View Media
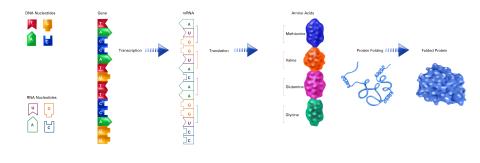
2510: From DNA to Protein (labeled)
2510: From DNA to Protein (labeled)
The genetic code in DNA is transcribed into RNA, which is translated into proteins with specific sequences. During transcription, nucleotides in DNA are copied into RNA, where they are read three at a time to encode the amino acids in a protein. Many parts of a protein fold as the amino acids are strung together.
See image 2509 for an unlabeled version of this illustration.
Featured in The Structures of Life.
See image 2509 for an unlabeled version of this illustration.
Featured in The Structures of Life.
Crabtree + Company
View Media
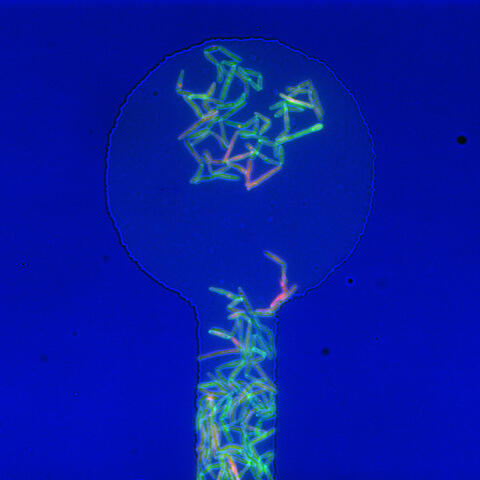
5751: Genetically identical mycobacteria respond differently to antibiotic 1
5751: Genetically identical mycobacteria respond differently to antibiotic 1
Antibiotic resistance in microbes is a serious health concern. So researchers have turned their attention to how bacteria undo the action of some antibiotics. Here, scientists set out to find the conditions that help individual bacterial cells survive in the presence of the antibiotic rifampicin. The research team used Mycobacterium smegmatis, a more harmless relative of Mycobacterium tuberculosis, which infects the lung and other organs and causes serious disease.
In this image, genetically identical mycobacteria are growing in a miniature growth chamber called a microfluidic chamber. Using live imaging, the researchers found that individual mycobacteria will respond differently to the antibiotic, depending on the growth stage and other timing factors. The researchers used genetic tagging with green fluorescent protein to distinguish cells that can resist rifampicin and those that cannot. With this gene tag, cells tolerant of the antibiotic light up in green and those that are susceptible in violet, enabling the team to monitor the cells' responses in real time.
To learn more about how the researchers studied antibiotic resistance in mycobacteria, see this news release from Tufts University. Related to video 5752.
In this image, genetically identical mycobacteria are growing in a miniature growth chamber called a microfluidic chamber. Using live imaging, the researchers found that individual mycobacteria will respond differently to the antibiotic, depending on the growth stage and other timing factors. The researchers used genetic tagging with green fluorescent protein to distinguish cells that can resist rifampicin and those that cannot. With this gene tag, cells tolerant of the antibiotic light up in green and those that are susceptible in violet, enabling the team to monitor the cells' responses in real time.
To learn more about how the researchers studied antibiotic resistance in mycobacteria, see this news release from Tufts University. Related to video 5752.
Bree Aldridge, Tufts University
View Media
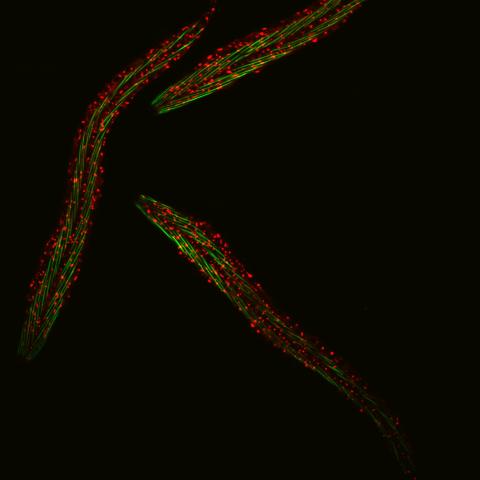
6582: Group of fluorescent C. elegans showing muscle and ribosomal protein
6582: Group of fluorescent C. elegans showing muscle and ribosomal protein
Three C. elegans, tiny roundworms, with a ribosomal protein glowing red and muscle fibers glowing green. Researchers used these worms to study a molecular pathway that affects aging. The ribosomal protein is involved in protein translation and may play a role in dietary restriction-induced longevity. Image created using confocal microscopy.
View single roundworm here 6581.
View closeup of roundworms here 6583.
View single roundworm here 6581.
View closeup of roundworms here 6583.
Jarod Rollins, Mount Desert Island Biological Laboratory.
View Media
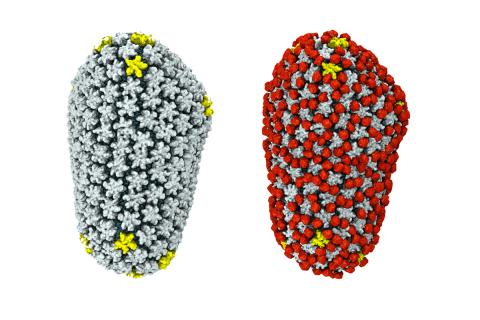
3755: Cryo-EM reveals how the HIV capsid attaches to a human protein to evade immune detection
3755: Cryo-EM reveals how the HIV capsid attaches to a human protein to evade immune detection
The illustration shows the capsid of human immunodeficiency virus (HIV) whose molecular features were resolved with cryo-electron microscopy (cryo-EM). On the left, the HIV capsid is "naked," a state in which it would be easily detected by and removed from cells. However, as shown on the right, when the viral capsid binds to and is covered with a host protein, called cyclophilin A (shown in red), it evades detection and enters and invades the human cell to use it to establish an infection. To learn more about how cyclophilin A helps HIV infect cells and how scientists used cryo-EM to find out the mechanism by which the HIV capsid attaches to cyclophilin A, see this news release by the University of Illinois. A study reporting these findings was published in the journal Nature Communications.
Juan R. Perilla, University of Illinois at Urbana-Champaign
View Media