Switch to List View
Image and Video Gallery
This is a searchable collection of scientific photos, illustrations, and videos. The images and videos in this gallery are licensed under Creative Commons Attribution Non-Commercial ShareAlike 3.0. This license lets you remix, tweak, and build upon this work non-commercially, as long as you credit and license your new creations under identical terms.
6556: Floral pattern in a mixture of two bacterial species, Acinetobacter baylyi and Escherichia coli, grown on a semi-solid agar for 72 hour
6556: Floral pattern in a mixture of two bacterial species, Acinetobacter baylyi and Escherichia coli, grown on a semi-solid agar for 72 hour
Floral pattern emerging as two bacterial species, motile Acinetobacter baylyi and non-motile Escherichia coli (green), are grown together for 72 hours on 0.5% agar surface from a small inoculum in the center of a Petri dish.
See 6557 for a photo of this process at 24 hours on 0.75% agar surface.
See 6553 for a photo of this process at 48 hours on 1% agar surface.
See 6555 for another photo of this process at 48 hours on 1% agar surface.
See 6550 for a video of this process.
See 6557 for a photo of this process at 24 hours on 0.75% agar surface.
See 6553 for a photo of this process at 48 hours on 1% agar surface.
See 6555 for another photo of this process at 48 hours on 1% agar surface.
See 6550 for a video of this process.
L. Xiong et al, eLife 2020;9: e48885
View Media
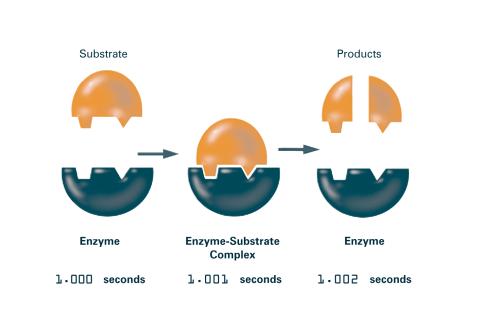
2522: Enzymes convert subtrates into products (with labels)
2522: Enzymes convert subtrates into products (with labels)
Enzymes convert substrates into products very quickly. See image 2521 for an unlabeled version of this illustration. Featured in The Chemistry of Health.
Crabtree + Company
View Media
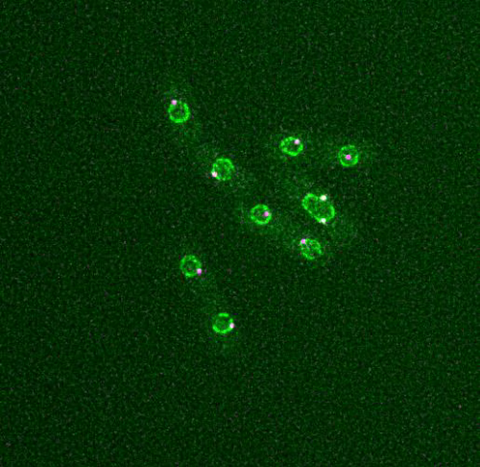
6795: Dividing yeast cells with nuclear envelopes and spindle pole bodies
6795: Dividing yeast cells with nuclear envelopes and spindle pole bodies
Time-lapse video of yeast cells undergoing cell division. Nuclear envelopes are shown in green, and spindle pole bodies, which help pull apart copied genetic information, are shown in magenta. This video was captured using wide-field microscopy with deconvolution.
Related to images 6791, 6792, 6793, 6794, 6797, 6798, and video 6796.
Related to images 6791, 6792, 6793, 6794, 6797, 6798, and video 6796.
Alaina Willet, Kathy Gould’s lab, Vanderbilt University.
View Media
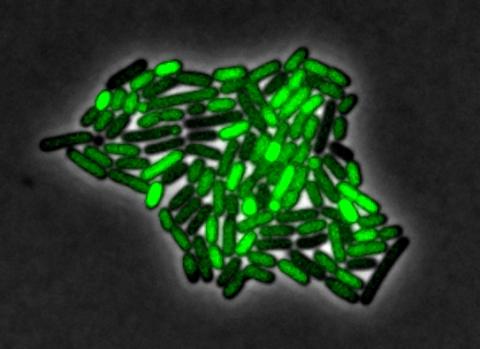
3253: Pulsating response to stress in bacteria
3253: Pulsating response to stress in bacteria
By attaching fluorescent proteins to the genetic circuit responsible for B. subtilis's stress response, researchers can observe the cells' pulses as green flashes. In response to a stressful environment like one lacking food, B. subtilis activates a large set of genes that help it respond to the hardship. Instead of leaving those genes on as previously thought, researchers discovered that the bacteria flip the genes on and off, increasing the frequency of these pulses with increasing stress. See entry 3254 for the related video.
Michael Elowitz, Caltech University
View Media
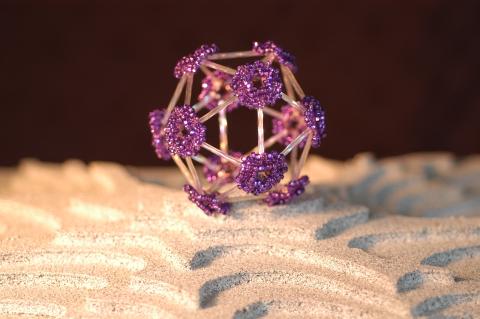
2305: Beaded bacteriophage
2305: Beaded bacteriophage
This sculpture made of purple and clear glass beads depicts bacteriophage Phi174, a virus that infects bacteria. It rests on a surface that portrays an adaptive landscape, a conceptual visualization. The ridges represent the gene combinations associated with the greatest fitness levels of the virus, as measured by how quickly the virus can reproduce itself. Phi174 is an important model system for studies of viral evolution because its genome can readily be sequenced as it evolves under defined laboratory conditions.
Holly Wichman, University of Idaho. (Surface by A. Johnston; photo by J. Palmersheim)
View Media
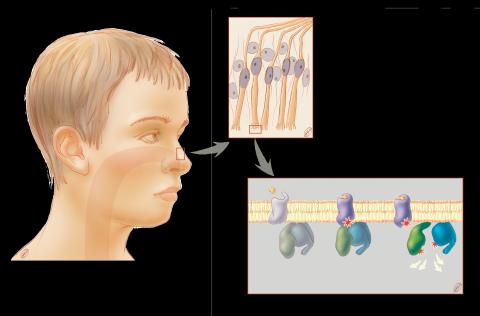
1291: Olfactory system
1291: Olfactory system
Sensory organs have cells equipped for detecting signals from the environment, such as odors. Receptors in the membranes of nerve cells in the nose bind to odor molecules, triggering a cascade of chemical reactions tranferred by G proteins into the cytoplasm.
Judith Stoffer
View Media
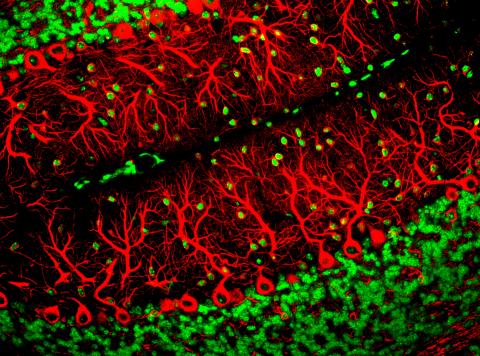
3637: Purkinje cells are one of the main cell types in the brain
3637: Purkinje cells are one of the main cell types in the brain
This image captures Purkinje cells (red), one of the main types of nerve cell found in the brain. These cells have elaborate branching structures called dendrites that receive signals from other nerve cells.
This image was part of the Life: Magnified exhibit that ran from June 3, 2014, to January 21, 2015, at Dulles International Airport.
This image was part of the Life: Magnified exhibit that ran from June 3, 2014, to January 21, 2015, at Dulles International Airport.
Yinghua Ma and Timothy Vartanian, Cornell University, Ithaca, N.Y.
View Media
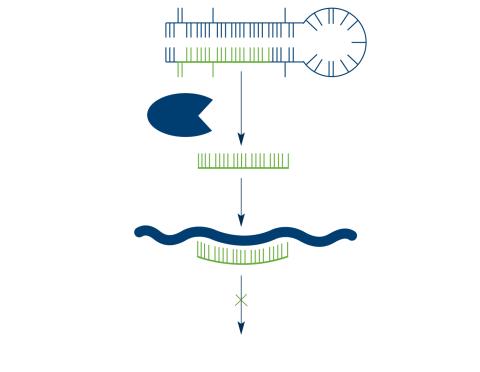
2556: Dicer generates microRNAs
2556: Dicer generates microRNAs
The enzyme Dicer generates microRNAs by chopping larger RNA molecules into tiny Velcro®-like pieces. MicroRNAs stick to mRNA molecules and prevent the mRNAs from being made into proteins. See image 2557 for a labeled version of this illustration. Featured in The New Genetics.
Crabtree + Company
View Media
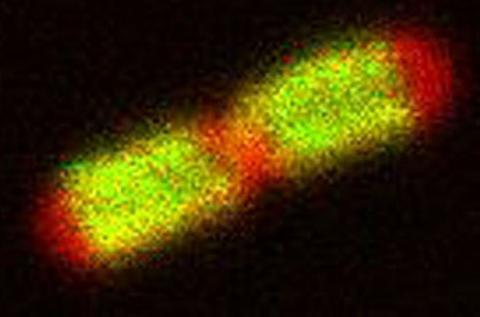
3549: TonB protein in gram-negative bacteria
3549: TonB protein in gram-negative bacteria
The green in this image highlights a protein called TonB, which is produced by many gram-negative bacteria, including those that cause typhoid fever, meningitis and dysentery. TonB lets bacteria take up iron from the host's body, which they need to survive. More information about the research behind this image can be found in a Biomedical Beat Blog posting from August 2013.
Phillip Klebba, Kansas State University
View Media
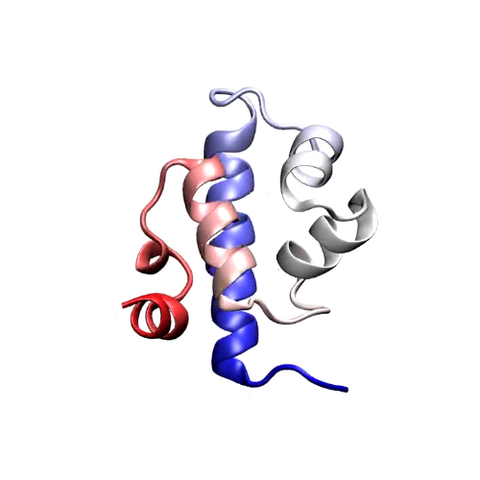
3391: Protein folding video
3391: Protein folding video
Proteins are long chains of amino acids. Each protein has a unique amino acid sequence. It is still a mystery how a protein folds into the proper shape based on its sequence. Scientists hope that one day they can "watch" this folding process for any given protein. The dream has been realized, at least partially, through the use of computer simulation.
Theoretical and Computational Biophysics Group
View Media
2740: Early life of a protein
2740: Early life of a protein
This illustration represents the early life of a protein—specifically, apomyoglobin—as it is synthesized by a ribosome and emerges from the ribosomal tunnel, which contains the newly formed protein's conformation. The synthesis occurs in the complex swirl of the cell medium, filled with interactions among many molecules. Researchers in Silvia Cavagnero's laboratory are studying the structure and dynamics of newly made proteins and polypeptides using spectroscopic and biochemical techniques.
Silvia Cavagnero, University of Wisconsin, Madison
View Media
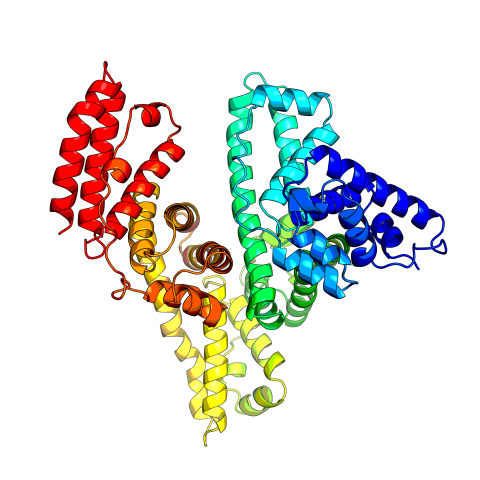
3745: Serum albumin structure 2
3745: Serum albumin structure 2
Serum albumin (SA) is the most abundant protein in the blood plasma of mammals. SA has a characteristic heart-shape structure and is a highly versatile protein. It helps maintain normal water levels in our tissues and carries almost half of all calcium ions in human blood. SA also transports some hormones, nutrients and metals throughout the bloodstream. Despite being very similar to our own SA, those from other animals can cause some mild allergies in people. Therefore, some scientists study SAs from humans and other mammals to learn more about what subtle structural or other differences cause immune responses in the body.
Related to entries 3744 and 3746
Related to entries 3744 and 3746
Wladek Minor, University of Virginia
View Media
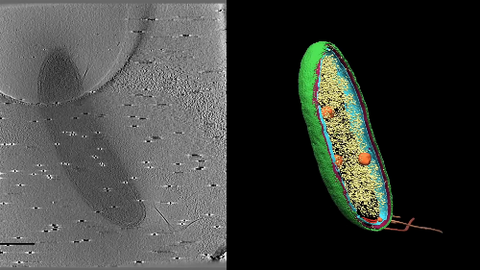
6569: Cryo-electron tomography of a Caulobacter bacterium
6569: Cryo-electron tomography of a Caulobacter bacterium
3D image of Caulobacter bacterium with various components highlighted: cell membranes (red and blue), protein shell (green), protein factories known as ribosomes (yellow), and storage granules (orange).
Peter Dahlberg, Stanford University.
View Media
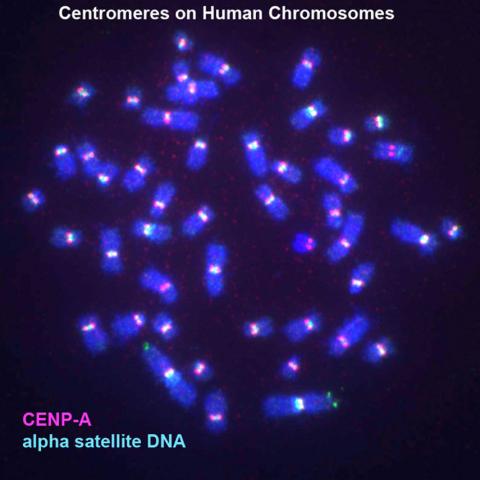
3255: Centromeres on human chromosomes
3255: Centromeres on human chromosomes
Human metaphase chromosomes are visible with fluorescence in vitro hybridization (FISH). Centromeric alpha satellite DNA (green) are found in the heterochromatin at each centromere. Immunofluorescence with CENP-A (red) shows the centromere-specific histone H3 variant that specifies the kinetochore.
Peter Warburton, Mount Sinai School of Medicine
View Media
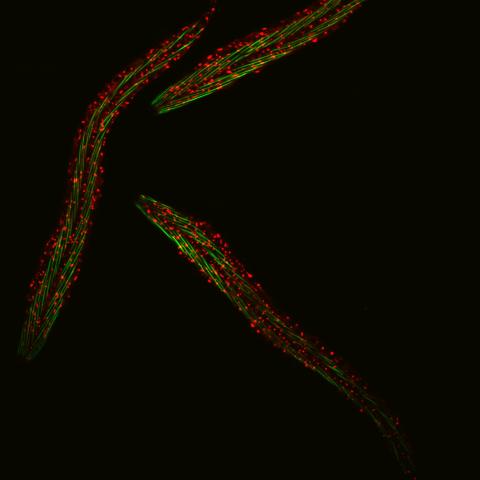
6582: Group of fluorescent C. elegans showing muscle and ribosomal protein
6582: Group of fluorescent C. elegans showing muscle and ribosomal protein
Three C. elegans, tiny roundworms, with a ribosomal protein glowing red and muscle fibers glowing green. Researchers used these worms to study a molecular pathway that affects aging. The ribosomal protein is involved in protein translation and may play a role in dietary restriction-induced longevity. Image created using confocal microscopy.
View single roundworm here 6581.
View closeup of roundworms here 6583.
View single roundworm here 6581.
View closeup of roundworms here 6583.
Jarod Rollins, Mount Desert Island Biological Laboratory.
View Media
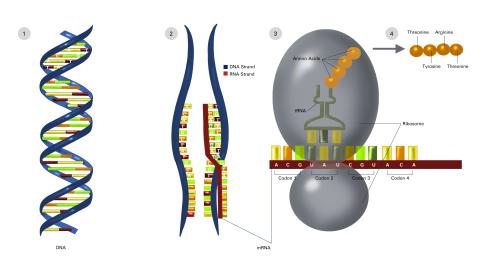
2549: Central dogma, illustrated (with labels and numbers for stages)
2549: Central dogma, illustrated (with labels and numbers for stages)
DNA encodes RNA, which encodes protein. DNA is transcribed to make messenger RNA (mRNA). The mRNA sequence (dark red strand) is complementary to the DNA sequence (blue strand). On ribosomes, transfer RNA (tRNA) reads three nucleotides at a time in mRNA to bring together the amino acids that link up to make a protein. See image 2548 for a version of this illustration that isn't numbered and 2547 for a an entirely unlabeled version. Featured in The New Genetics.
Crabtree + Company
View Media
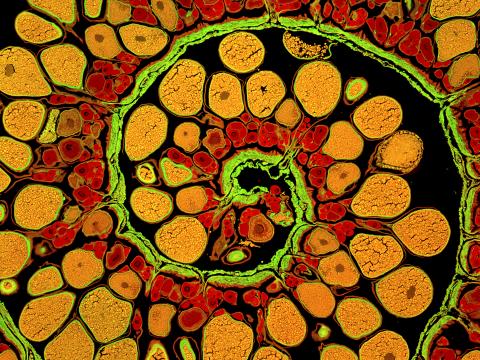
3620: Anglerfish ovary cross-section
3620: Anglerfish ovary cross-section
This image captures the spiral-shaped ovary of an anglerfish in cross-section. Once matured, these eggs will be released in a gelatinous, floating mass. For some species of anglerfish, this egg mass can be up to 3 feet long and include nearly 200,000 eggs.
This image was part of the Life: Magnified exhibit that ran from June 3, 2014, to January 21, 2015, at Dulles International Airport.
This image was part of the Life: Magnified exhibit that ran from June 3, 2014, to January 21, 2015, at Dulles International Airport.
James E. Hayden, The Wistar Institute, Philadelphia, Pa.
View Media
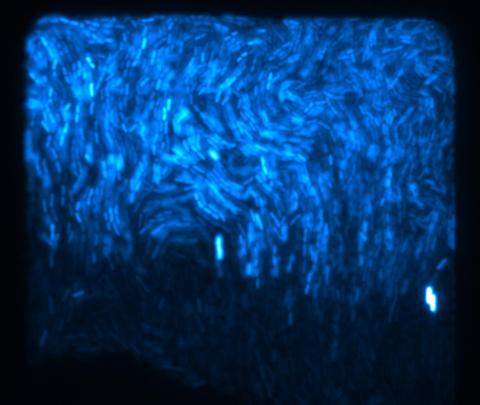
3268: Fluorescent E. coli bacteria
3268: Fluorescent E. coli bacteria
Bioengineers were able to coax bacteria to blink in unison on microfluidic chips. They called each blinking bacterial colony a biopixel. Thousands of fluorescent E. coli bacteria, shown here, make up a biopixel. Related to images 3265 and 3266. From a UC San Diego news release, "Researchers create living 'neon signs' composed of millions of glowing bacteria."
Jeff Hasty Lab, UC San Diego
View Media
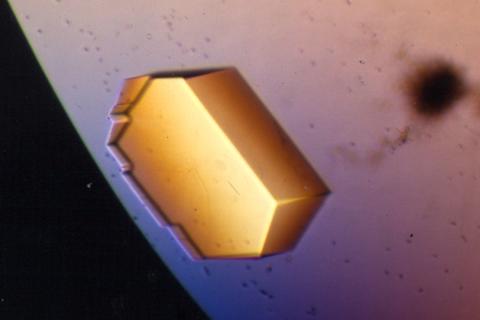
2413: Pig trypsin (2)
2413: Pig trypsin (2)
A crystal of porcine trypsin protein created for X-ray crystallography, which can reveal detailed, three-dimensional protein structures.
Alex McPherson, University of California, Irvine
View Media
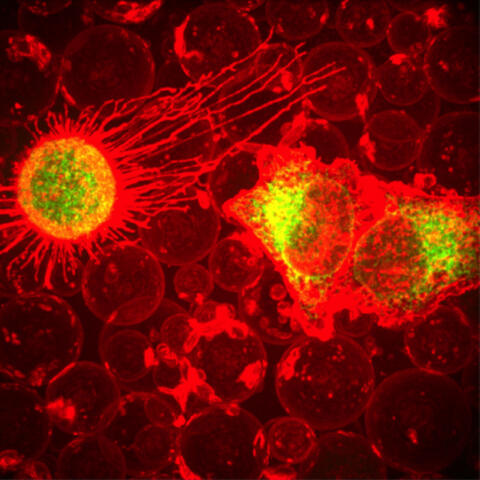
5887: Plasma-Derived Membrane Vesicles
5887: Plasma-Derived Membrane Vesicles
This fiery image doesn’t come from inside a bubbling volcano. Instead, it shows animal cells caught in the act of making bubbles, or blebbing. Some cells regularly pinch off parts of their membranes to produce bubbles filled with a mix of proteins and fats. The bubbles (red) are called plasma-derived membrane vesicles, or PMVs, and can travel to other parts of the body where they may aid in cell-cell communication. The University of Texas, Austin, researchers responsible for this photo are exploring ways to use PMVs to deliver medicines to precise locations in the body.
This image, entered in the Biophysical Society’s 2017 Art of Science Image contest, used two-channel spinning disk confocal fluorescence microscopy. It was also featured in the NIH Director’s Blog in May 2017.
This image, entered in the Biophysical Society’s 2017 Art of Science Image contest, used two-channel spinning disk confocal fluorescence microscopy. It was also featured in the NIH Director’s Blog in May 2017.
Jeanne Stachowiak, University of Texas at Austin
View Media
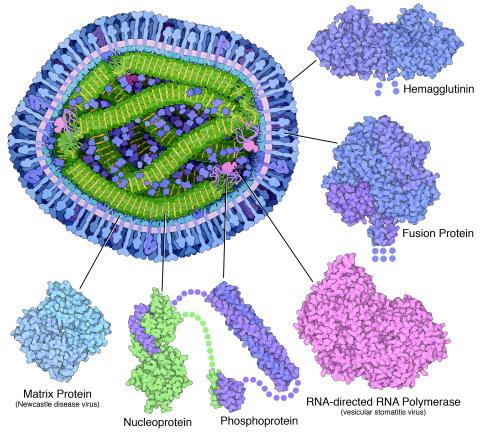
6996: Measles virus proteins
6996: Measles virus proteins
A cross section of the measles virus in which six proteins (enlarged on the outside of the virus) work together to infect cells. The measles virus is extremely infectious; 9 out of 10 people exposed will contract the disease. Fortunately, an effective vaccine protects against infection. Portions of the proteins that have not been determined are shown with dots.
Learn more about the six proteins on PDB 101’s Molecule of the Month: Measles Virus Proteins. Structures are available for the ordered regions of nucleoprotein and phosphoprotein (PDB entries 5E4V, 3ZDO, 1T6O), but the remaining regions are thought to form a flexible, random tangle. For a larger look at the measles virus, see 6995.
Learn more about the six proteins on PDB 101’s Molecule of the Month: Measles Virus Proteins. Structures are available for the ordered regions of nucleoprotein and phosphoprotein (PDB entries 5E4V, 3ZDO, 1T6O), but the remaining regions are thought to form a flexible, random tangle. For a larger look at the measles virus, see 6995.
Amy Wu and Christine Zardecki, RCSB Protein Data Bank.
View Media
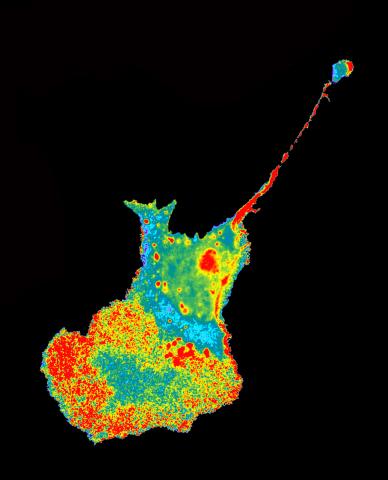
2454: Seeing signaling protein activation in cells 04
2454: Seeing signaling protein activation in cells 04
Cdc42, a member of the Rho family of small guanosine triphosphatase (GTPase) proteins, regulates multiple cell functions, including motility, proliferation, apoptosis, and cell morphology. In order to fulfill these diverse roles, the timing and location of Cdc42 activation must be tightly controlled. Klaus Hahn and his research group use special dyes designed to report protein conformational changes and interactions, here in living neutrophil cells. Warmer colors in this image indicate higher levels of activation. Cdc42 looks to be activated at cell protrusions.
Related to images 2451, 2452, and 2453.
Related to images 2451, 2452, and 2453.
Klaus Hahn, University of North Carolina, Chapel Hill Medical School
View Media

3344: Artificial cilia exhibit spontaneous beating
3344: Artificial cilia exhibit spontaneous beating
Researchers have created artificial cilia that wave like the real thing. Zvonimir Dogic and his Brandeis University colleagues combined just a few cilia proteins to create cilia that are able to wave and sweep material around--although more slowly and simply than real ones. The researchers are using the lab-made cilia to study how the structures coordinate their movements and what happens when they don't move properly. Featured in the August 18, 2011, issue of Biomedical Beat.
Zvonimir Dogic
View Media
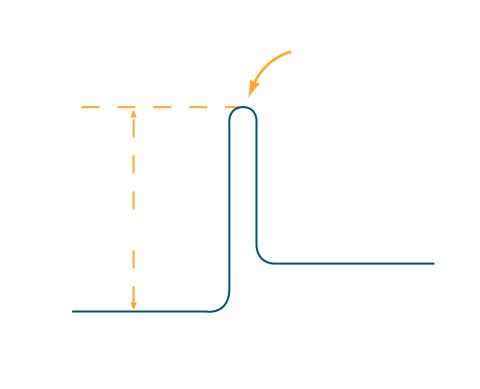
2525: Activation energy
2525: Activation energy
To become products, reactants must overcome an energy hill. See image 2526 for a labeled version of this illustration. Featured in The Chemistry of Health.
Crabtree + Company
View Media
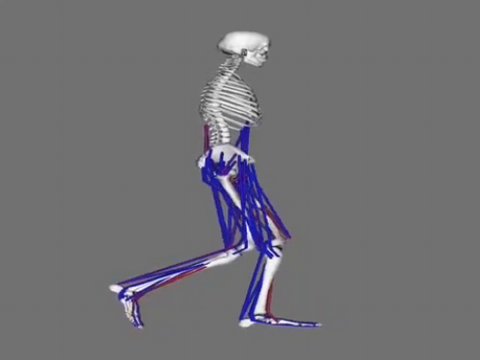
6598: Simulation of leg muscles moving
6598: Simulation of leg muscles moving
When we walk, muscles and nerves interact in intricate ways. This simulation, which is based on data from a six-foot-tall man, shows these interactions.
Chand John and Eran Guendelman, Stanford University
View Media
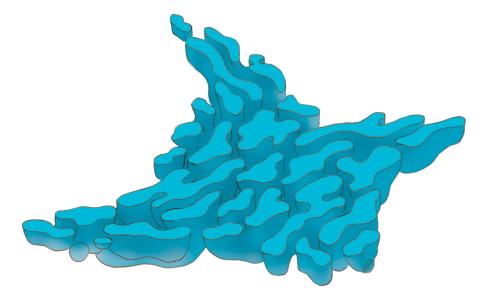
1292: Smooth ER
1292: Smooth ER
The endoplasmic reticulum comes in two types: Rough ER is covered with ribosomes and prepares newly made proteins; smooth ER specializes in making lipids and breaking down toxic molecules.
Judith Stoffer
View Media
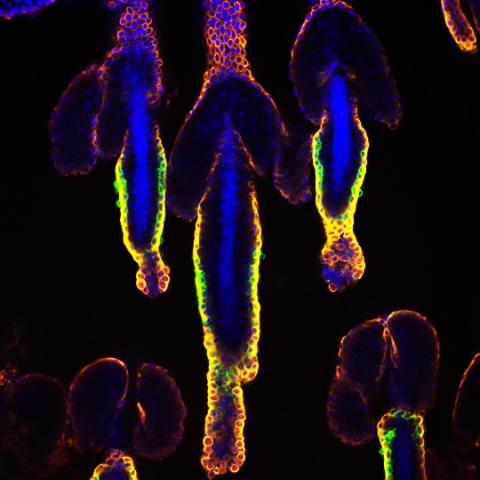
3500: Wound healing in process
3500: Wound healing in process
Wound healing requires the action of stem cells. In mice that lack the Sept2/ARTS gene, stem cells involved in wound healing live longer and wounds heal faster and more thoroughly than in normal mice. This confocal microscopy image from a mouse lacking the Sept2/ARTS gene shows a tail wound in the process of healing. See more information in the article in Science.
Related to images 3497 and 3498.
Related to images 3497 and 3498.
Hermann Steller, Rockefeller University
View Media
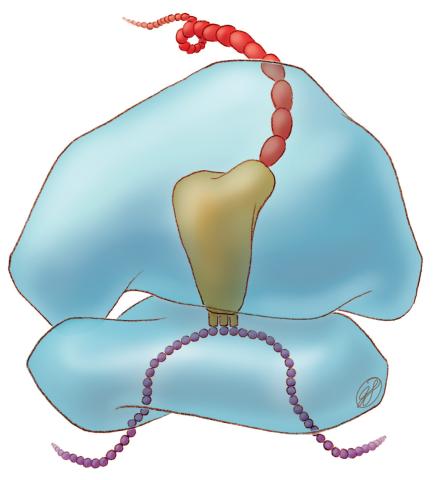
1281: Translation
1281: Translation
Ribosomes manufacture proteins based on mRNA instructions. Each ribosome reads mRNA, recruits tRNA molecules to fetch amino acids, and assembles the amino acids in the proper order.
Judith Stoffer
View Media
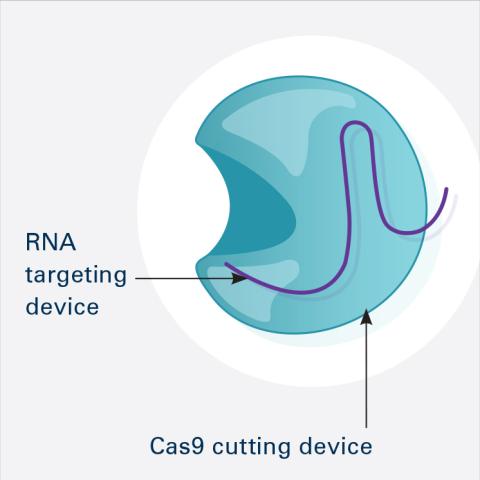
6465: CRISPR Illustration Frame 1
6465: CRISPR Illustration Frame 1
This illustration shows, in simplified terms, how the CRISPR-Cas9 system can be used as a gene-editing tool. This is the first frame in a series of four. The CRISPR system has two components joined together: a finely tuned targeting device (a small strand of RNA programmed to look for a specific DNA sequence) and a strong cutting device (an enzyme called Cas9 that can cut through a double strand of DNA).
For an explanation and overview of the CRISPR-Cas9 system, see the iBiology video, and find the full CRIPSR illustration here.
For an explanation and overview of the CRISPR-Cas9 system, see the iBiology video, and find the full CRIPSR illustration here.
National Institute of General Medical Sciences.
View Media
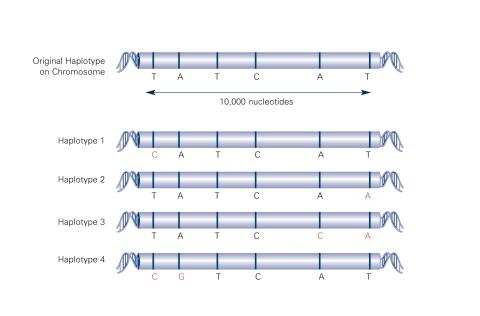
2567: Haplotypes (with labels)
2567: Haplotypes (with labels)
Haplotypes are combinations of gene variants that are likely to be inherited together within the same chromosomal region. In this example, an original haplotype (top) evolved over time to create three newer haplotypes that each differ by a few nucleotides (red). See image 2566 for an unlabeled version of this illustration. Featured in The New Genetics.
Crabtree + Company
View Media
2763: Fused, dicentric chromosomes
2763: Fused, dicentric chromosomes
This fused chromosome has two functional centromeres, shown as two sets of red and green dots. Centromeres are DNA/protein complexes that are key to splitting the chromosomes evenly during cell division. When dicentric chromosomes like this one are formed in a person, fertility problems or other difficulties may arise. Normal chromosomes carrying a single centromere (one set of red and green dots) are also visible in this image.
Beth A. Sullivan, Duke University
View Media
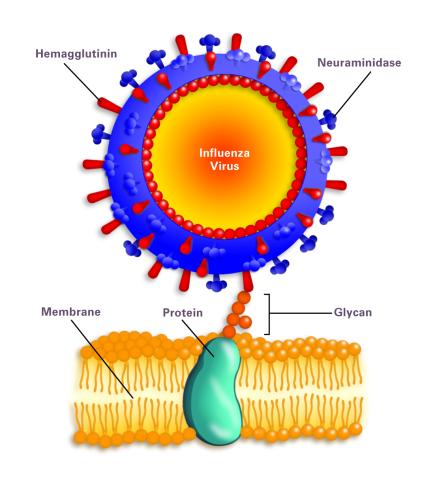
2505: Influenza virus attaches to host membrane (with labels)
2505: Influenza virus attaches to host membrane (with labels)
Influenza A infects a host cell when hemagglutinin grips onto glycans on its surface. Neuraminidase, an enzyme that chews sugars, helps newly made virus particles detach so they can infect other cells. Related to 213.
Crabtree + Company
View Media
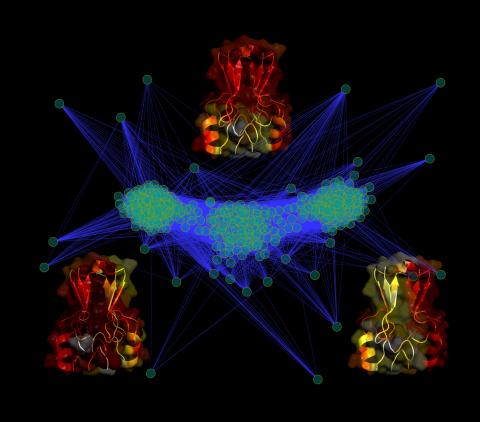
3295: Cluster analysis of mysterious protein
3295: Cluster analysis of mysterious protein
Researchers use cluster analysis to study protein shape and function. Each green circle represents one potential shape of the protein mitoNEET. The longer the blue line between two circles, the greater the differences between the shapes. Most shapes are similar; they fall into three clusters that are represented by the three images of the protein. From a Rice University news release. Graduate student Elizabeth Baxter and Patricia Jennings, professor of chemistry and biochemistry at UCSD, collaborated with José Onuchic, a physicist at Rice University, on this work.
Patricia Jennings and Elizabeth Baxter, University of California, San Diego
View Media
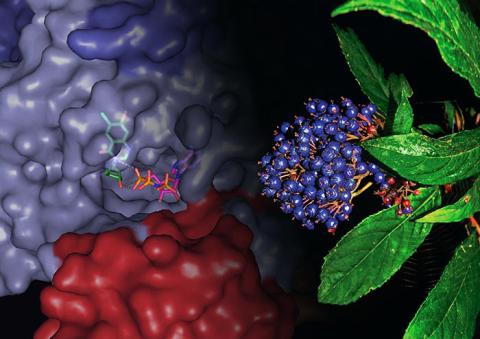
3483: Chang Shan
3483: Chang Shan
For thousands of years, Chinese herbalists have treated malaria using Chang Shan, a root extract from a type of hydrangea that grows in Tibet and Nepal. Recent studies have suggested Chang Shan can also reduce scar formation, treat multiple sclerosis and even slow cancer progression.
Paul Schimmel Lab, Scripps Research Institute
View Media
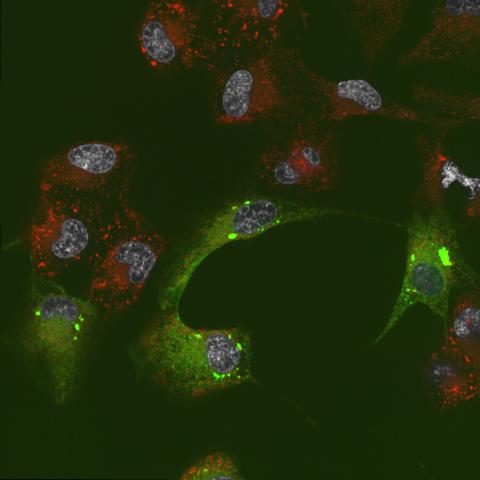
6774: Endoplasmic reticulum abnormalities 2
6774: Endoplasmic reticulum abnormalities 2
Human cells with the gene that codes for the protein FIT2 deleted. After an experimental intervention, they are expressing a nonfunctional version of FIT2, shown in green. The lack of functional FIT2 affected the structure of the endoplasmic reticulum (ER), and the nonfunctional protein clustered in ER membrane aggregates, seen as large bright-green spots. Lipid droplets are shown in red, and the nucleus is visible in gray. This image was captured using a confocal microscope. Related to image 6773.
Michel Becuwe, Harvard University.
View Media
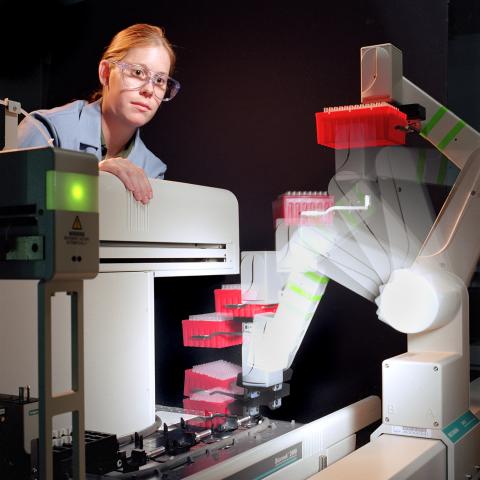
2356: Student overseeing protein cloning robot
2356: Student overseeing protein cloning robot
Student Christina Hueneke of the Midwest Center for Structural Genomics is overseeing a protein cloning robot. The robot was designed as part of an effort to exponentially increase the output of a traditional wet lab. Part of the center's goal is to cut the average cost of analyzing a protein from $200,000 to $20,000 and to slash the average time from months to days and hours.
Midwest Center for Structural Genomics
View Media

1014: Lily mitosis 04
1014: Lily mitosis 04
A light microscope image of a cell from the endosperm of an African globe lily (Scadoxus katherinae). This is one frame of a time-lapse sequence that shows cell division in action. The lily is considered a good organism for studying cell division because its chromosomes are much thicker and easier to see than human ones. Staining shows microtubules in red and chromosomes in blue.
Related to images 1010, 1011, 1012, 1013, 1015, 1016, 1017, 1018, 1019, and 1021.
Related to images 1010, 1011, 1012, 1013, 1015, 1016, 1017, 1018, 1019, and 1021.
Andrew S. Bajer, University of Oregon, Eugene
View Media
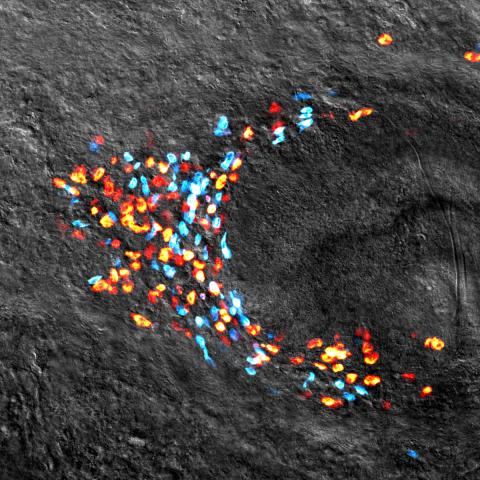
3306: Planarian stem cell colony
3306: Planarian stem cell colony
Planarians are freshwater flatworms that have powerful abilities to regenerate their bodies, which would seem to make them natural model organisms in which to study stem cells. But until recently, scientists had not been able to efficiently find the genes that regulate the planarian stem cell system. In this image, a single stem cell has given rise to a colony of stem cells in a planarian. Proliferating cells are red, and differentiating cells are blue. Quantitatively measuring the size and ratios of these two cell types provides a powerful framework for studying the roles of stem cell regulatory genes in planarians.
Peter Reddien, Whitehead Institute
View Media
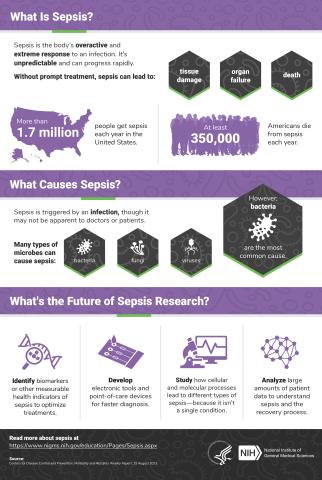
6536: Sepsis Infographic
6536: Sepsis Infographic
Sepsis is the body’s overactive and extreme response to an infection. More than 1.7 million people get sepsis each year in the United States. Without prompt treatment, sepsis can lead to tissue damage, organ failure, and death. Many NIGMS-supported researchers are working to improve sepsis diagnosis and treatment. Learn more with our sepsis featured topic page.
See 6551 for the Spanish version of this infographic.
See 6551 for the Spanish version of this infographic.
National Institute of General Medical Sciences
View Media
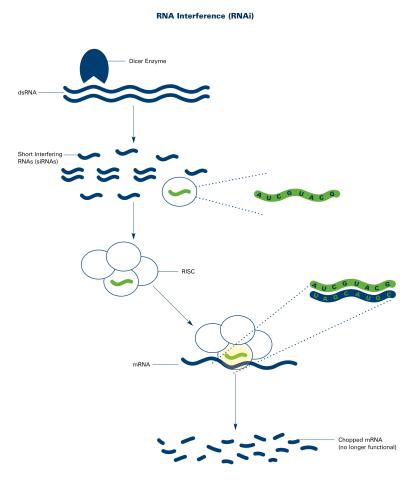
2559: RNA interference (with labels)
2559: RNA interference (with labels)
RNA interference or RNAi is a gene-silencing process in which double-stranded RNAs trigger the destruction of specific RNAs. See 2558 for an unlabeled version of this illustration. Featured in The New Genetics.
Crabtree + Company
View Media
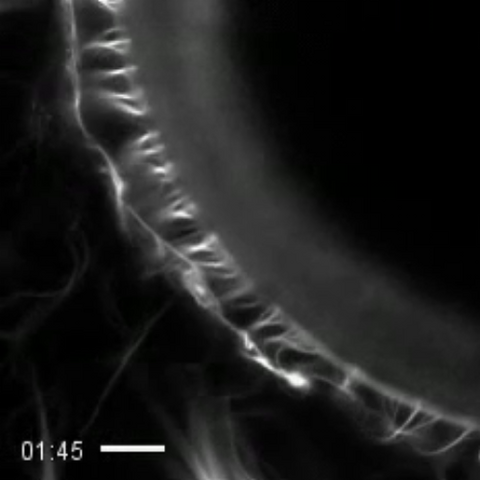
3494: How cilia do the wave
3494: How cilia do the wave
Thin, hair-like biological structures called cilia are tiny but mighty. Each one, made up of more than 600 different proteins, works together with hundreds of others in a tightly-packed layer to move like a crowd at a ball game doing "the wave." Their synchronized motion helps sweep mucus from the lungs and usher eggs from the ovaries into the uterus. By controlling how fluid flows around an embryo, cilia also help ensure that organs like the heart develop on the correct side of your body.
Zvonimir Dogic, Brandeis University
View Media
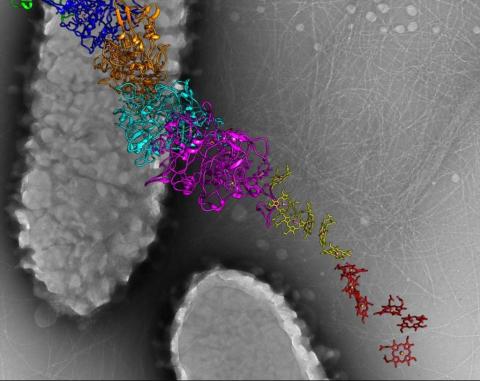
6580: Bacterial nanowire model
6580: Bacterial nanowire model
A model of a Geobacter sulfurreducens nanowire created from cryo-electron microscopy images. The bacterium conducts electricity through these nanowires, which are made up of protein and iron-containing molecules.
Edward Egelman, University of Virginia.
View Media
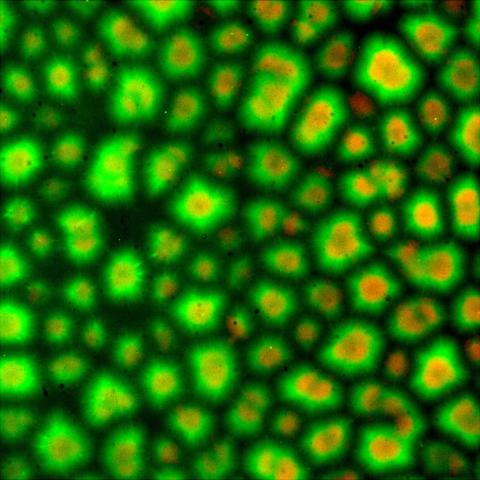
6586: Cell-like compartments from frog eggs 3
6586: Cell-like compartments from frog eggs 3
Cell-like compartments that spontaneously emerged from scrambled frog eggs. Endoplasmic reticulum (red) and microtubules (green) are visible. Image created using epifluorescence microscopy.
For more photos of cell-like compartments from frog eggs view: 6584, 6585, 6591, 6592, and 6593.
For videos of cell-like compartments from frog eggs view: 6587, 6588, 6589, and 6590.
Xianrui Cheng, Stanford University School of Medicine.
View Media
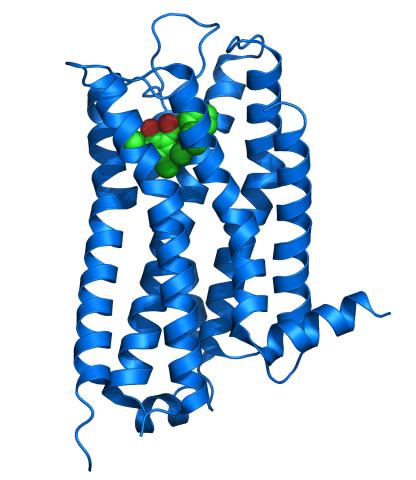
3363: Dopamine D3 receptor
3363: Dopamine D3 receptor
The receptor is shown bound to an antagonist, eticlopride
Raymond Stevens, The Scripps Research Institute
View Media
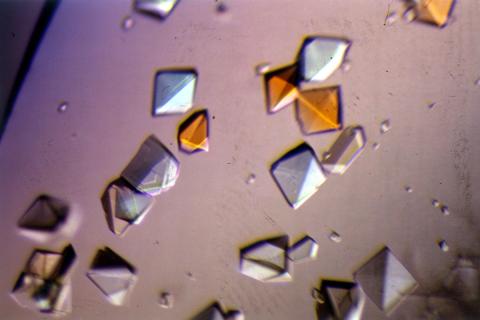
2412: Pig alpha amylase
2412: Pig alpha amylase
Crystals of porcine alpha amylase protein created for X-ray crystallography, which can reveal detailed, three-dimensional protein structures.
Alex McPherson, University of California, Irvine
View Media
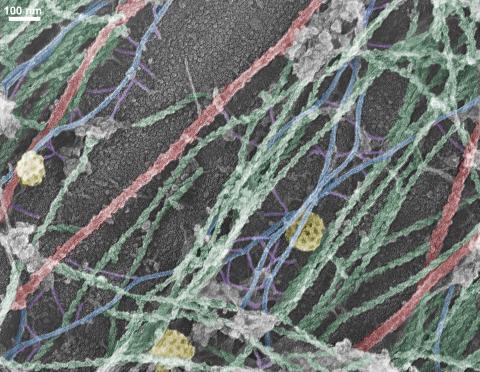
3634: Cells use bubble-like structures called vesicles to transport cargo
3634: Cells use bubble-like structures called vesicles to transport cargo
Cells use bubble-like structures called vesicles (yellow) to import, transport, and export cargo and in cellular communication. A single cell may be filled with thousands of moving vesicles.
This image was part of the Life: Magnified exhibit that ran from June 3, 2014, to January 21, 2015, at Dulles International Airport.
This image was part of the Life: Magnified exhibit that ran from June 3, 2014, to January 21, 2015, at Dulles International Airport.
Tatyana Svitkina, University of Pennsylvania
View Media
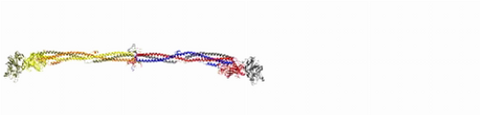
2450: Blood clots show their flex
2450: Blood clots show their flex
Blood clots stop bleeding, but they also can cause heart attacks and strokes. A team led by computational biophysicist Klaus Schulten of the University of Illinois at Urbana-Champaign has revealed how a blood protein can give clots their lifesaving and life-threatening abilities. The researchers combined experimental and computational methods to animate fibrinogen, a protein that forms the elastic fibers that enable clots to withstand the force of blood pressure. This simulation shows that the protein, through a series of events, stretches up to three times its length. Adjusting this elasticity could improve how we manage healthful and harmful clots. NIH's National Center for Research Resources also supported this work. Featured in the March 19, 2008, issue of Biomedical Beat.
Eric Lee, University of Illinois at Urbana-Champaign
View Media
2432: ARTS triggers apoptosis
2432: ARTS triggers apoptosis
Cell showing overproduction of the ARTS protein (red). ARTS triggers apoptosis, as shown by the activation of caspase-3 (green) a key tool in the cell's destruction. The nucleus is shown in blue. Image is featured in October 2015 Biomedical Beat blog post Cool Images: A Halloween-Inspired Cell Collection.
Hermann Steller, Rockefeller University
View Media