Switch to List View
Image and Video Gallery
This is a searchable collection of scientific photos, illustrations, and videos. The images and videos in this gallery are licensed under Creative Commons Attribution Non-Commercial ShareAlike 3.0. This license lets you remix, tweak, and build upon this work non-commercially, as long as you credit and license your new creations under identical terms.
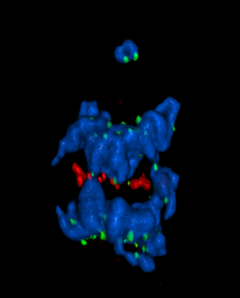
5766: A chromosome goes missing in anaphase
5766: A chromosome goes missing in anaphase
Anaphase is the critical step during mitosis when sister chromosomes are disjoined and directed to opposite spindle poles, ensuring equal distribution of the genome during cell division. In this image, one pair of sister chromosomes at the top was lost and failed to divide after chemical inhibition of polo-like kinase 1. This image depicts chromosomes (blue) separating away from the spindle mid-zone (red). Kinetochores (green) highlight impaired movement of some chromosomes away from the mid-zone or the failure of sister chromatid separation (top). Scientists are interested in detailing the signaling events that are disrupted to produce this effect. The image is a volume projection of multiple deconvolved z-planes acquired with a Nikon widefield fluorescence microscope.
This image was chosen as a winner of the 2016 NIH-funded research image call. The research that led to this image was funded by NIGMS.
Related to image 5765.
View Media
This image was chosen as a winner of the 2016 NIH-funded research image call. The research that led to this image was funded by NIGMS.
Related to image 5765.
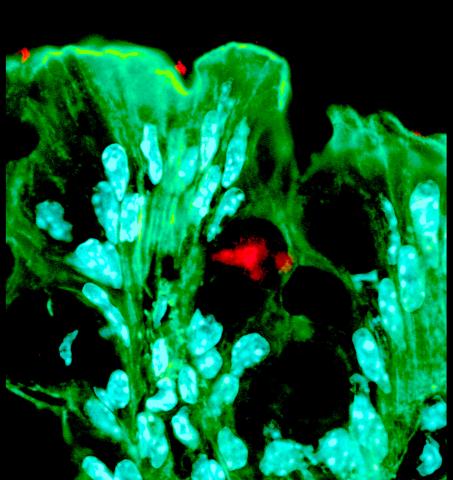
3527: Bacteria in the mouse colon
3527: Bacteria in the mouse colon
Image of the colon of a mouse mono-colonized with Bacteroides fragilis (red) residing within the crypt channel. The red staining is due to an antibody to B. fragilis, the green staining is a general dye for the mouse cells (phalloidin, which stains F-actin) and the light blue glow is from a dye for visualizing the mouse cell nuclei (DAPI, which stains DNA). Bacteria from the human microbiome have evolved specific molecules to physically associate with host tissue, conferring resilience and stability during life-long colonization of the gut. Image is featured in October 2015 Biomedical Beat blog post Cool Images: A Halloween-Inspired Cell Collection.
Sarkis K. Mazmanian, California Institute of Technology
View Media
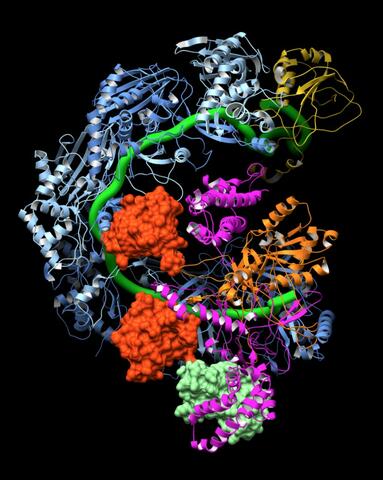
6352: CRISPR surveillance complex
6352: CRISPR surveillance complex
This image shows how the CRISPR surveillance complex is disabled by two copies of anti-CRISPR protein AcrF1 (red) and one AcrF2 (light green). These anti-CRISPRs block access to the CRISPR RNA (green tube) preventing the surveillance complex from scanning and targeting invading viral DNA for destruction.
NRAMM National Resource for Automated Molecular Microscopy http://nramm.nysbc.org/nramm-images/ Source: Bridget Carragher
View Media
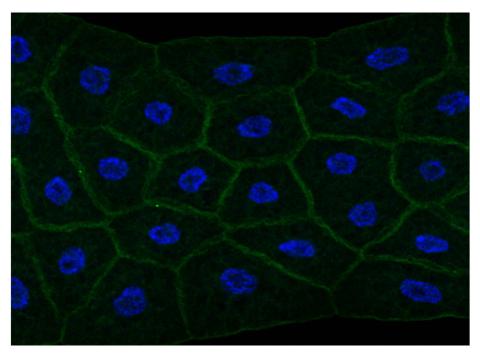
2757: Draper, shown in the fatbody of a Drosophila melanogaster larva
2757: Draper, shown in the fatbody of a Drosophila melanogaster larva
The fly fatbody is a nutrient storage and mobilization organ akin to the mammalian liver. The engulfment receptor Draper (green) is located at the cell surface of fatbody cells. The cell nuclei are shown in blue.
Christina McPhee and Eric Baehrecke, University of Massachusetts Medical School
View Media
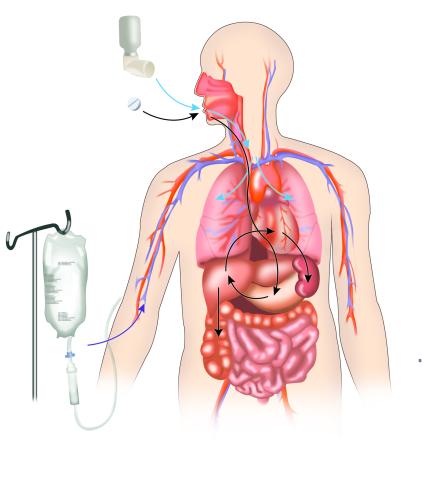
2527: A drug's life in the body
2527: A drug's life in the body
A drug's life in the body. Medicines taken by mouth pass through the liver before they are absorbed into the bloodstream. Other forms of drug administration bypass the liver, entering the blood directly. See 2528 for a labeled version of this illustration. Featured in Medicines By Design.
Crabtree + Company
View Media
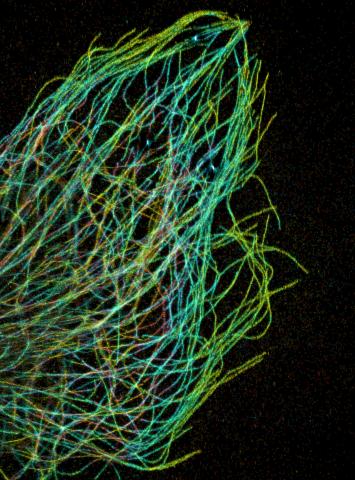
3611: Tiny strands of tubulin, a protein in a cell's skeleton
3611: Tiny strands of tubulin, a protein in a cell's skeleton
Just as our bodies rely on bones for structural support, our cells rely on a cellular skeleton. In addition to helping cells keep their shape, this cytoskeleton transports material within cells and coordinates cell division. One component of the cytoskeleton is a protein called tubulin, shown here as thin strands.
This image was part of the Life: Magnified exhibit that ran from June 3, 2014, to January 21, 2015, at Dulles International Airport.
This image was part of the Life: Magnified exhibit that ran from June 3, 2014, to January 21, 2015, at Dulles International Airport.
Pakorn Kanchanawong, National University of Singapore and National Heart, Lung, and Blood Institute, National Institutes of Health; and Clare Waterman, National Heart, Lung, and Blood Institute, National Institutes of Health
View Media
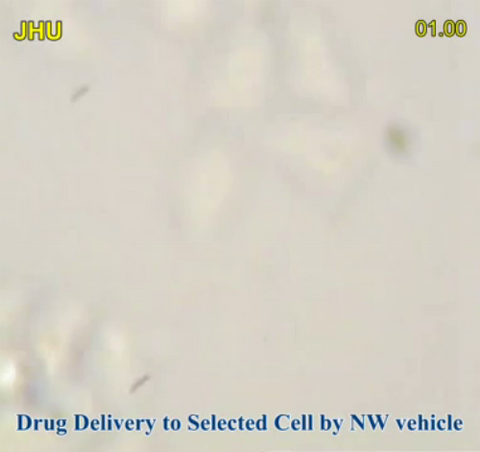
3779: Precisely Delivering Chemical Cargo to Cells
3779: Precisely Delivering Chemical Cargo to Cells
Moving protein or other molecules to specific cells to treat or examine them has been a major biological challenge. Scientists have now developed a technique for delivering chemicals to individual cells. The approach involves gold nanowires that, for example, can carry tumor-killing proteins. The advance was possible after researchers developed electric tweezers that could manipulate gold nanowires to help deliver drugs to single cells.
This movie shows the manipulation of the nanowires for drug delivery to a single cell. To learn more about this technique, see this post in the Computing Life series.
This movie shows the manipulation of the nanowires for drug delivery to a single cell. To learn more about this technique, see this post in the Computing Life series.
Nature Nanotechnology
View Media
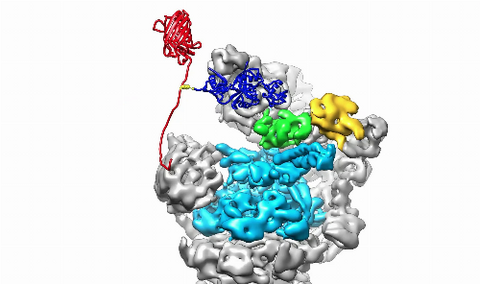
3764: Movie of the 19S proteasome subunit processing a protein substrate
3764: Movie of the 19S proteasome subunit processing a protein substrate
The proteasome is a critical multiprotein complex in the cell that breaks down and recycles proteins that have become damaged or are no longer needed. This movie shows how a protein substrate (red) is bound through its ubiquitin chain (blue) to one of the ubiquitin receptors of the proteasome (Rpn10, yellow). The substrate's flexible engagement region then gets engaged by the AAA+ motor of the proteasome (cyan), which initiates mechanical pulling, unfolding and movement of the protein into the proteasome's interior for cleavage into shorter protein pieces called peptides. During movement of the substrate, its ubiquitin modification gets cleaved off by the deubiquitinase Rpn11 (green), which sits directly above the entrance to the AAA+ motor pore and acts as a gatekeeper to ensure efficient ubiquitin removal, a prerequisite for fast protein breakdown by the 26S proteasome. Related to image 3763.
Andreas Martin, HHMI
View Media
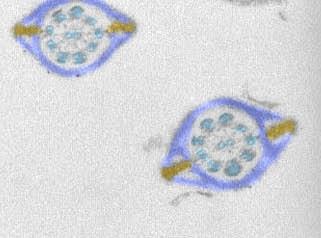
1191: Mouse sperm sections
1191: Mouse sperm sections
This transmission electron micrograph shows sections of mouse sperm tails, or flagella.
Tina Weatherby Carvalho, University of Hawaii at Manoa
View Media
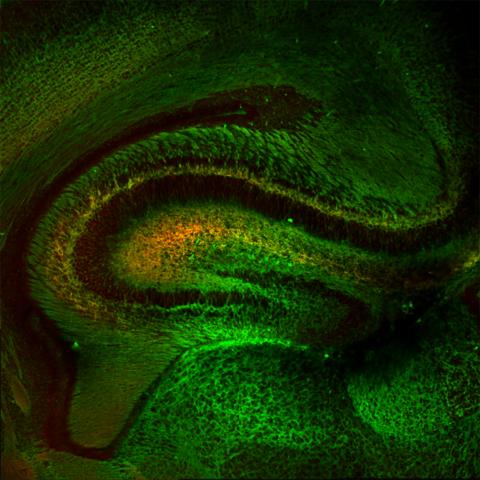
5886: Mouse Brain Cross Section
5886: Mouse Brain Cross Section
The brain sections are treated with fluorescent antibodies specific to a particular protein and visualized using serial electron microscopy (SEM).
Anton Maximov, The Scripps Research Institute, La Jolla, CA
View Media
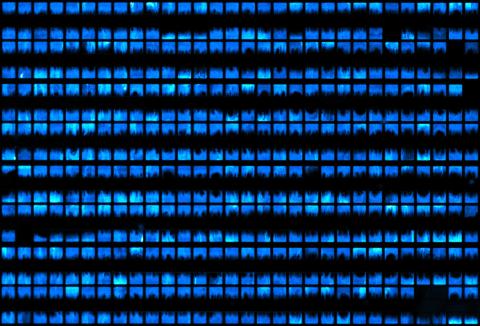
3266: Biopixels
3266: Biopixels
Bioengineers were able to coax bacteria to blink in unison on microfluidic chips. This image shows a small chip with about 500 blinking bacterial colonies or biopixels. Related to images 3265 and 3268. From a UC San Diego news release, "Researchers create living 'neon signs' composed of millions of glowing bacteria."
Jeff Hasty Lab, UC San Diego
View Media
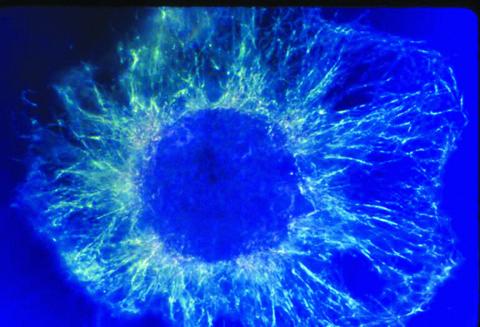
1058: Lily mitosis 01
1058: Lily mitosis 01
A light microscope image shows the chromosomes, stained dark blue, in a dividing cell of an African globe lily (Scadoxus katherinae). This is one frame of a time-lapse sequence that shows cell division in action. The lily is considered a good organism for studying cell division because its chromosomes are much thicker and easier to see than human ones.
Andrew S. Bajer, University of Oregon, Eugene
View Media
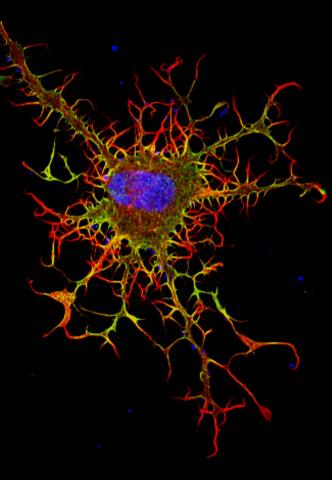
3613: Abnormal, spiky fibroblast
3613: Abnormal, spiky fibroblast
This is a fibroblast, a connective tissue cell that plays an important role in wound healing. Normal fibroblasts have smooth edges. In contrast, this spiky cell is missing a protein that is necessary for proper construction of the cell's skeleton. Its jagged shape makes it impossible for the cell to move normally. In addition to compromising wound healing, abnormal cell movement can lead to birth defects, faulty immune function, and other health problems.
This image was part of the Life: Magnified exhibit that ran from June 3, 2014, to January 21, 2015, at Dulles International Airport.
This image was part of the Life: Magnified exhibit that ran from June 3, 2014, to January 21, 2015, at Dulles International Airport.
Praveen Suraneni, Stowers Institute for Medical Research, Kansas City, Mo.
View Media
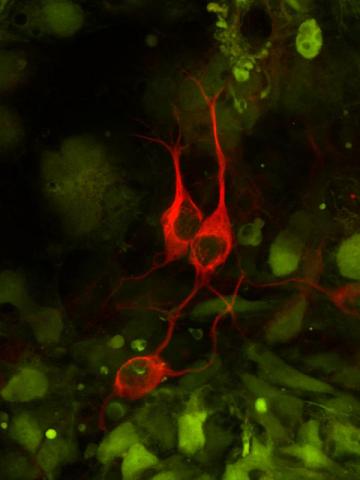
3290: Three neurons and human ES cells
3290: Three neurons and human ES cells
The three neurons (red) visible in this image were derived from human embryonic stem cells. Undifferentiated stem cells are green here. Image and caption information courtesy of the California Institute for Regenerative Medicine.
Anirvan Ghosh lab, University of California, San Diego, via CIRM
View Media
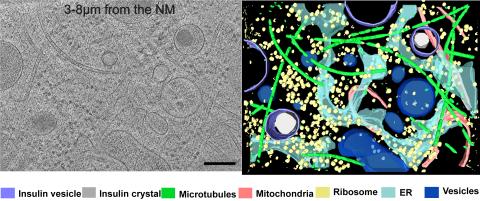
6608: Cryo-ET cross-section of a rat pancreas cell
6608: Cryo-ET cross-section of a rat pancreas cell
On the left, a cross-section slice of a rat pancreas cell captured using cryo-electron tomography (cryo-ET). On the right, a 3D, color-coded version of the image highlighting cell structures. Visible features include microtubules (neon-green rods), ribosomes (small yellow circles), and vesicles (dark-blue circles). These features are surrounded by the partially visible endoplasmic reticulum (light blue). The black line at the bottom right of the left image represents 200 nm. Related to image 6607.
Xianjun Zhang, University of Southern California.
View Media
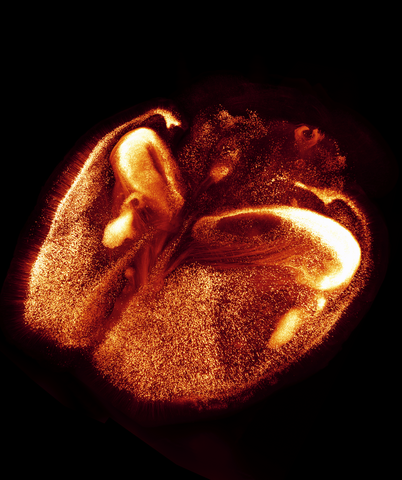
6930: Mouse brain 2
6930: Mouse brain 2
A mouse brain that was genetically modified so that subpopulations of its neurons glow. Researchers often study mice because they share many genes with people and can shed light on biological processes, development, and diseases in humans.
This image was captured using a light sheet microscope.
Related to image 6929 and video 6931.
This image was captured using a light sheet microscope.
Related to image 6929 and video 6931.
Prayag Murawala, MDI Biological Laboratory and Hannover Medical School.
View Media
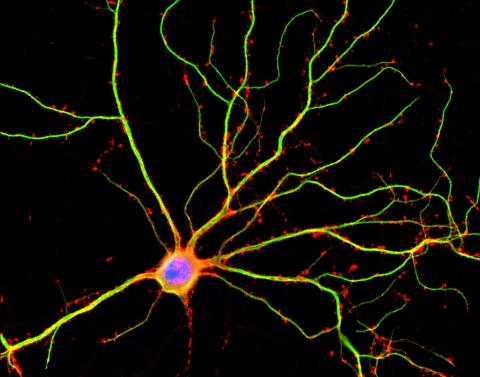
3687: Hippocampal neuron in culture
3687: Hippocampal neuron in culture
Hippocampal neuron in culture. Dendrites are green, dendritic spines are red and DNA in cell's nucleus is blue. Image is featured on Biomedical Beat blog post Anesthesia and Brain Cells: A Temporary Disruption?
Shelley Halpain, UC San Diego
View Media
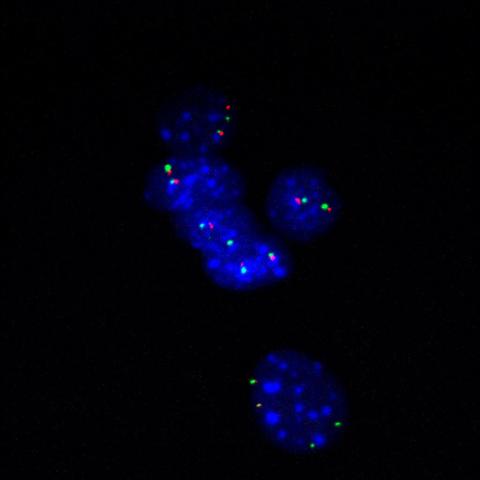
3296: Fluorescence in situ hybridization (FISH) in mouse ES cells shows DNA interactions
3296: Fluorescence in situ hybridization (FISH) in mouse ES cells shows DNA interactions
Researchers used fluorescence in situ hybridization (FISH) to confirm the presence of long range DNA-DNA interactions in mouse embryonic stem cells. Here, two loci labeled in green (Oct4) and red that are 13 Mb apart on linear DNA are frequently found to be in close proximity. DNA-DNA colocalizations like this are thought to both reflect and contribute to cell type specific gene expression programs.
Kathrin Plath, University of California, Los Angeles
View Media
2740: Early life of a protein
2740: Early life of a protein
This illustration represents the early life of a protein—specifically, apomyoglobin—as it is synthesized by a ribosome and emerges from the ribosomal tunnel, which contains the newly formed protein's conformation. The synthesis occurs in the complex swirl of the cell medium, filled with interactions among many molecules. Researchers in Silvia Cavagnero's laboratory are studying the structure and dynamics of newly made proteins and polypeptides using spectroscopic and biochemical techniques.
Silvia Cavagnero, University of Wisconsin, Madison
View Media
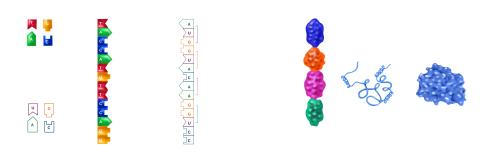
2509: From DNA to Protein
2509: From DNA to Protein
Nucleotides in DNA are copied into RNA, where they are read three at a time to encode the amino acids in a protein. Many parts of a protein fold as the amino acids are strung together.
See image 2510 for a labeled version of this illustration.
Featured in The Structures of Life.
See image 2510 for a labeled version of this illustration.
Featured in The Structures of Life.
Crabtree + Company
View Media
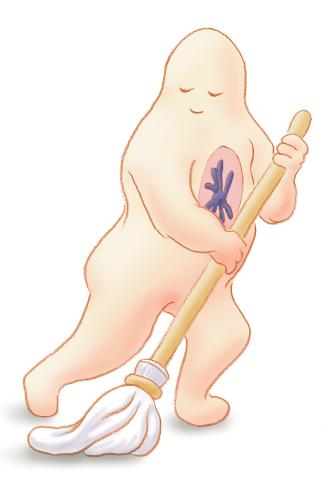
1311: Housekeeping cell illustration
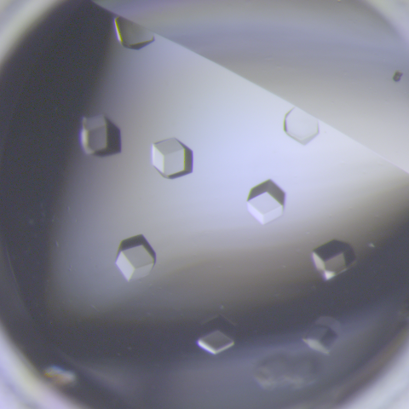
6764: Crystals of CCD-1 in complex with cefotaxime
6764: Crystals of CCD-1 in complex with cefotaxime
CCD-1 is an enzyme produced by the bacterium Clostridioides difficile that helps it resist antibiotics. Here, researchers crystallized bound pairs of CCD-1 molecules and molecules of the antibiotic cefotaxime. This enabled their structure to be studied using X-ray crystallography.
Related to images 6765, 6766, and 6767.
Related to images 6765, 6766, and 6767.
Keith Hodgson, Stanford University.
View Media
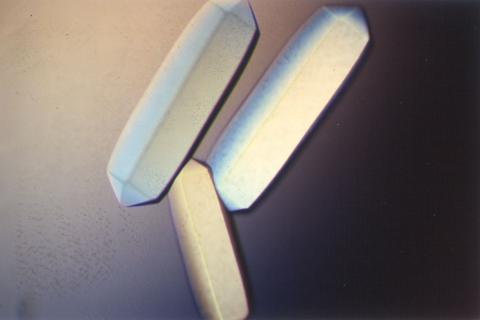
2411: Fungal lipase (2)
2411: Fungal lipase (2)
Crystals of fungal lipase protein created for X-ray crystallography, which can reveal detailed, three-dimensional protein structures.
Alex McPherson, University of California, Irvine
View Media
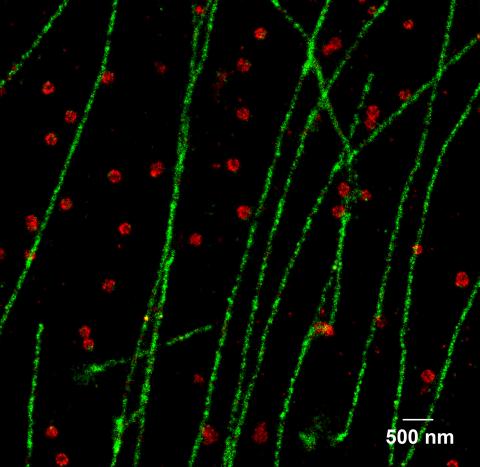
2325: Multicolor STORM
2325: Multicolor STORM
In 2006, scientists developed an optical microscopy technique enabling them to clearly see individual molecules within cells. In 2007, they took the technique, abbreviated STORM, a step further. They identified multicolored probes that let them peer into cells and clearly see multiple cellular components at the same time, such as these microtubules (green) and small hollows called clathrin-coated pits (red). Unlike conventional methods, the multicolor STORM technique produces a crisp and high resolution picture. A sharper view of how cellular components interact will likely help scientists answer some longstanding questions about cell biology.
Xiaowei Zhuang, Harvard University
View Media
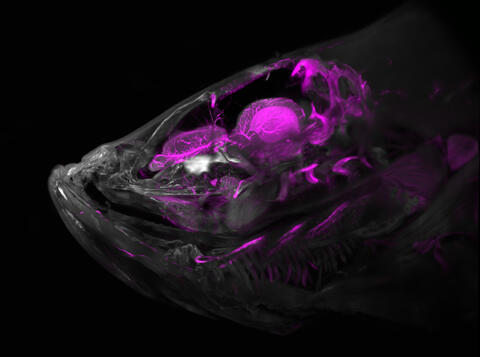
6934: Zebrafish head vasculature
6934: Zebrafish head vasculature
A zebrafish head with blood vessels shown in purple. Researchers often study zebrafish because they share many genes with humans, grow and reproduce quickly, and have see-through eggs and embryos, which make it easy to study early stages of development.
This image was captured using a light sheet microscope.
Related to video 6933.
This image was captured using a light sheet microscope.
Related to video 6933.
Prayag Murawala, MDI Biological Laboratory and Hannover Medical School.
View Media
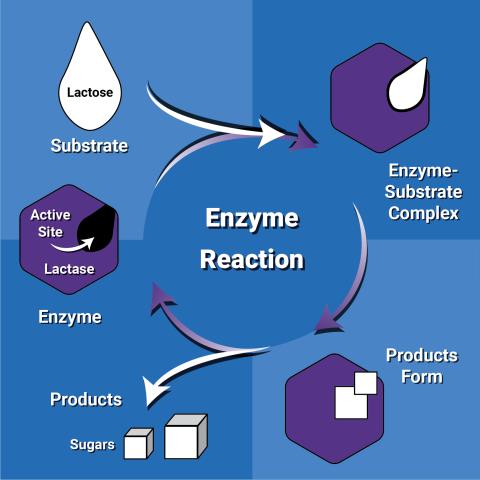
6604: Enzyme reaction
6604: Enzyme reaction
Enzymes speed up chemical reactions by reducing the amount of energy needed for the reactions. The substrate (lactose) binds to the active site of the enzyme (lactase) and is converted into products (sugars).
NIGMS
View Media
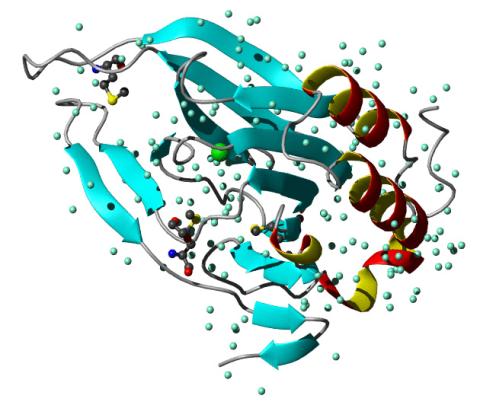
2347: Cysteine dioxygenase from mouse
2347: Cysteine dioxygenase from mouse
Model of the mammalian iron enzyme cysteine dioxygenase from a mouse.
Center for Eukaryotic Structural Genomics, PSI
View Media
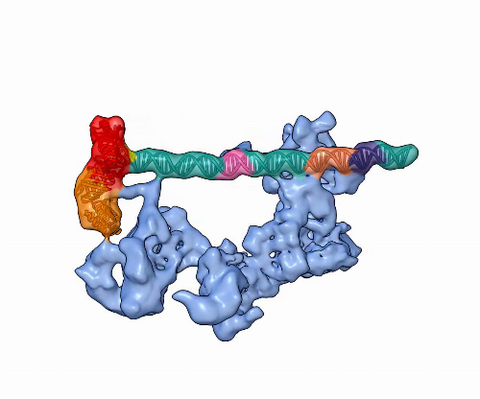
5730: Dynamic cryo-EM model of the human transcription preinitiation complex
5730: Dynamic cryo-EM model of the human transcription preinitiation complex
Gene transcription is a process by which information encoded in DNA is transcribed into RNA. It's essential for all life and requires the activity of proteins, called transcription factors, that detect where in a DNA strand transcription should start. In eukaryotes (i.e., those that have a nucleus and mitochondria), a protein complex comprising 14 different proteins is responsible for sniffing out transcription start sites and starting the process. This complex represents the core machinery to which an enzyme, named RNA polymerase, can bind to and read the DNA and transcribe it to RNA. Scientists have used cryo-electron microscopy (cryo-EM) to visualize the TFIID-RNA polymerase-DNA complex in unprecedented detail. This animation shows the different TFIID components as they contact DNA and recruit the RNA polymerase for gene transcription.
To learn more about the research that has shed new light on gene transcription, see this news release from Berkeley Lab.
Related to image 3766.
To learn more about the research that has shed new light on gene transcription, see this news release from Berkeley Lab.
Related to image 3766.
Eva Nogales, Berkeley Lab
View Media
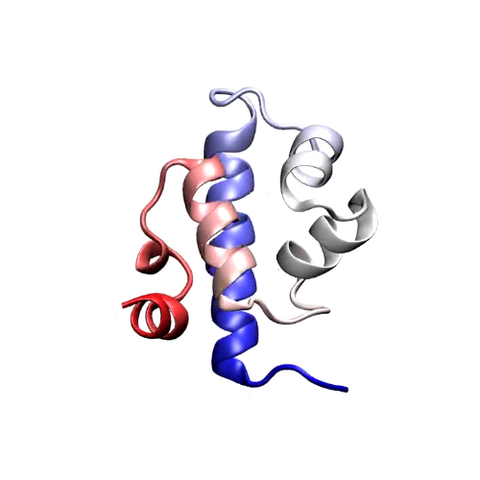
3391: Protein folding video
3391: Protein folding video
Proteins are long chains of amino acids. Each protein has a unique amino acid sequence. It is still a mystery how a protein folds into the proper shape based on its sequence. Scientists hope that one day they can "watch" this folding process for any given protein. The dream has been realized, at least partially, through the use of computer simulation.
Theoretical and Computational Biophysics Group
View Media
2432: ARTS triggers apoptosis
2432: ARTS triggers apoptosis
Cell showing overproduction of the ARTS protein (red). ARTS triggers apoptosis, as shown by the activation of caspase-3 (green) a key tool in the cell's destruction. The nucleus is shown in blue. Image is featured in October 2015 Biomedical Beat blog post Cool Images: A Halloween-Inspired Cell Collection.
Hermann Steller, Rockefeller University
View Media
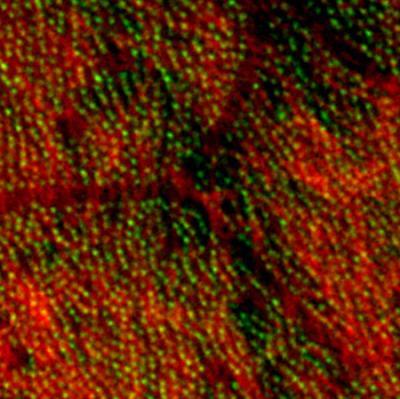
3292: Centrioles anchor cilia in planaria
3292: Centrioles anchor cilia in planaria
Centrioles (green) anchor cilia (red), which project on the surface of pharynx cells of the freshwater planarian Schmidtea mediterranea. Centrioles require cellular structures called centrosomes for assembly in other animal species, but this flatworm known for its regenerative ability was unexpectedly found to lack centrosomes. From a Stowers University news release.
Juliette Azimzadeh, University of California, San Francisco
View Media
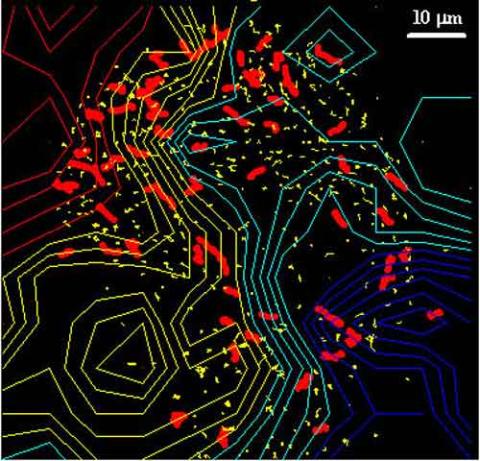
2310: Cellular traffic
2310: Cellular traffic
Like tractor-trailers on a highway, small sacs called vesicles transport substances within cells. This image tracks the motion of vesicles in a living cell. The short red and yellow marks offer information on vesicle movement. The lines spanning the image show overall traffic trends. Typically, the sacs flow from the lower right (blue) to the upper left (red) corner of the picture. Such maps help researchers follow different kinds of cellular processes as they unfold.
Alexey Sharonov and Robin Hochstrasser, University of Pennsylvania
View Media
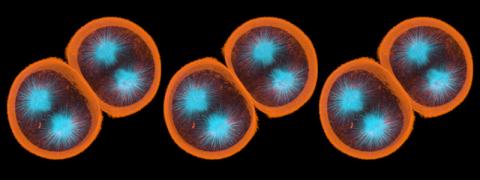
1047: Sea urchin embryo 01
1047: Sea urchin embryo 01
Stereo triplet of a sea urchin embryo stained to reveal actin filaments (orange) and microtubules (blue). This image is part of a series of images: image 1048, image 1049, image 1050, image 1051 and image 1052.
George von Dassow, University of Washington
View Media
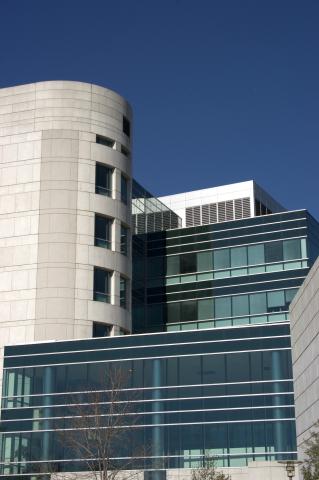
1089: Natcher Building 09
1089: Natcher Building 09
NIGMS staff are located in the Natcher Building on the NIH campus.
Alisa Machalek, National Institute of General Medical Sciences
View Media
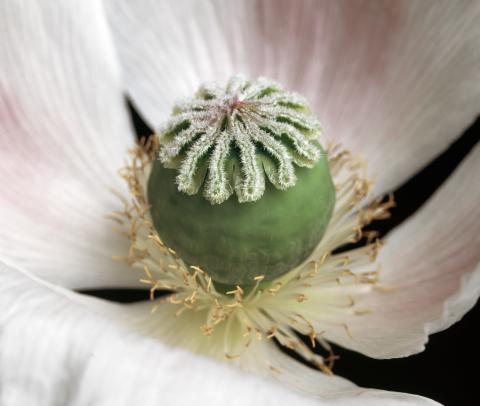
3423: White Poppy (cropped)
3423: White Poppy (cropped)
A cropped image of a white poppy. View poppy uncropped here 3424.
Judy Coyle, Donald Danforth Plant Science Center
View Media
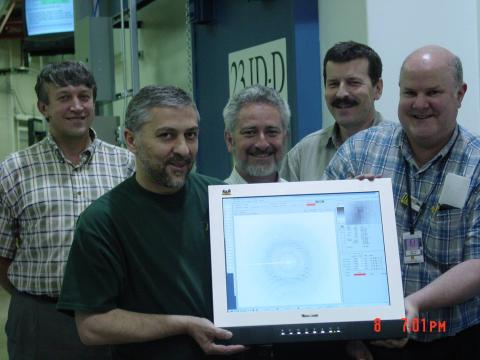
2384: Scientists display X-ray diffraction pattern obtained with split X-ray beamline
2384: Scientists display X-ray diffraction pattern obtained with split X-ray beamline
Scientists from Argonne National Laboratory's Advanced Photon Source (APS) display the first X-ray diffraction pattern obtained from a protein crystal using a split X-ray beam, the first of its kind at APS. The scientists shown are (from left to right): Oleg Makarov, Ruslan Sanishvili, Robert Fischetti (project manager), Sergey Stepanov, and Ward Smith.
GM/CA Collaborative Access Team
View Media
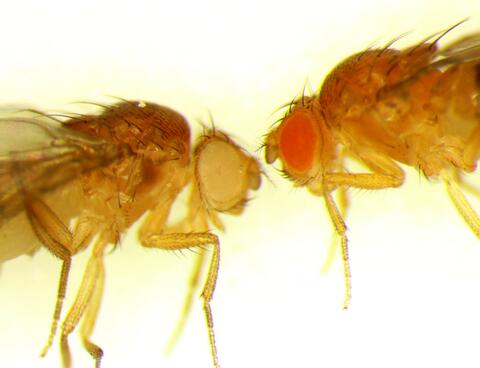
6344: Drosophila
6344: Drosophila
Two adult fruit flies (Drosophila)
Dr. Vicki Losick, MDI Biological Laboratory, www.mdibl.org
View Media
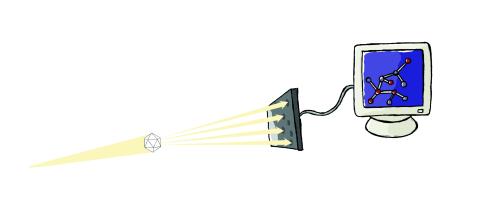
2511: X-ray crystallography
2511: X-ray crystallography
X-ray crystallography allows researchers to see structures too small to be seen by even the most powerful microscopes. To visualize the arrangement of atoms within molecules, researchers can use the diffraction patterns obtained by passing X-ray beams through crystals of the molecule. This is a common way for solving the structures of proteins. See image 2512 for a labeled version of this illustration. Featured in The Structures of Life.
Crabtree + Company
View Media
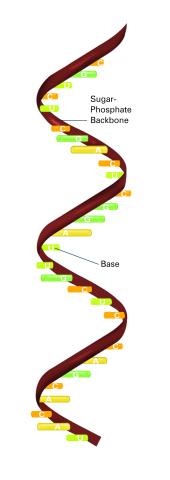
2555: RNA strand (with labels)
2555: RNA strand (with labels)
Ribonucleic acid (RNA) has a sugar-phosphate backbone and the bases adenine (A), cytosine (C), guanine (G), and uracil (U). Featured in The New Genetics.
See image 2554 for an unlabeled version of this illustration.
See image 2554 for an unlabeled version of this illustration.
Crabtree + Company
View Media
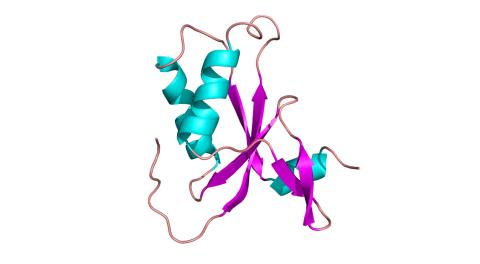
3427: Antitoxin GhoS (Illustration 1)
3427: Antitoxin GhoS (Illustration 1)
Structure of the bacterial antitoxin protein GhoS. GhoS inhibits the production of a bacterial toxin, GhoT, which can contribute to antibiotic resistance. GhoS is the first known bacterial antitoxin that works by cleaving the messenger RNA that carries the instructions for making the toxin. More information can be found in the paper: Wang X, Lord DM, Cheng HY, Osbourne DO, Hong SH, Sanchez-Torres V, Quiroga C, Zheng K, Herrmann T, Peti W, Benedik MJ, Page R, Wood TK. A new type V toxin-antitoxin system where mRNA for toxin GhoT is cleaved by antitoxin GhoS. Nat Chem Biol. 2012 Oct;8(10):855-61. Related to 3428.
Rebecca Page and Wolfgang Peti, Brown University and Thomas K. Wood, Pennsylvania State University
View Media
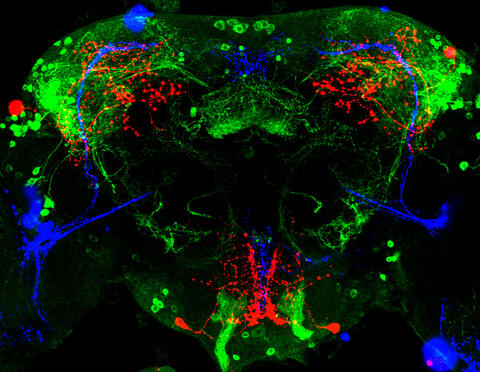
3754: Circadian rhythm neurons in the fruit fly brain
3754: Circadian rhythm neurons in the fruit fly brain
Some nerve cells (neurons) in the brain keep track of the daily cycle. This time-keeping mechanism, called the circadian clock, is found in all animals including us. The circadian clock controls our daily activities such as sleep and wakefulness. Researchers are interested in finding the neuron circuits involved in this time keeping and how the information about daily time in the brain is relayed to the rest of the body. In this image of a brain of the fruit fly Drosophila the time-of-day information flowing through the brain has been visualized by staining the neurons involved: clock neurons (shown in blue) function as "pacemakers" by communicating with neurons that produce a short protein called leucokinin (LK) (red), which, in turn, relays the time signal to other neurons, called LK-R neurons (green). This signaling cascade set in motion by the pacemaker neurons helps synchronize the fly's daily activity with the 24-hour cycle. To learn more about what scientists have found out about circadian pacemaker neurons in the fruit fly see this news release by New York University. This work was featured in the Biomedical Beat blog post Cool Image: A Circadian Circuit.
Justin Blau, New York University
View Media
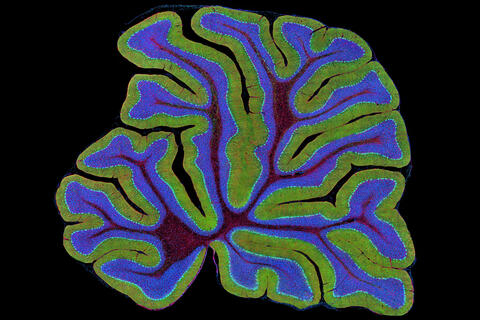
3723: Fluorescent microscopy of kidney tissue
3723: Fluorescent microscopy of kidney tissue
Serum albumin (SA) is the most abundant protein in the blood plasma of mammals. SA has a characteristic heart-shape structure and is a highly versatile protein. It helps maintain normal water levels in our tissues and carries almost half of all calcium ions in human blood. SA also transports some hormones, nutrients and metals throughout the bloodstream. Despite being very similar to our own SA, those from other animals can cause some mild allergies in people. Therefore, some scientists study SAs from humans and other mammals to learn more about what subtle structural or other differences cause immune responses in the body.
Related to entries 3725 and 3675.
Related to entries 3725 and 3675.
Tom Deerinck , National Center for Microscopy and Imaging Research
View Media
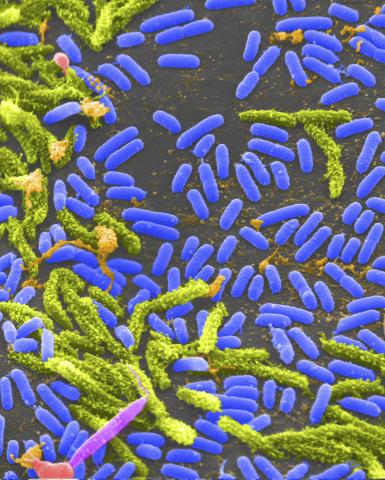
1160: Vibrio bacteria
1160: Vibrio bacteria
Vibrio, a type (genus) of rod-shaped bacteria. Some Vibrio species cause cholera in humans.
Tina Weatherby Carvalho, University of Hawaii at Manoa
View Media
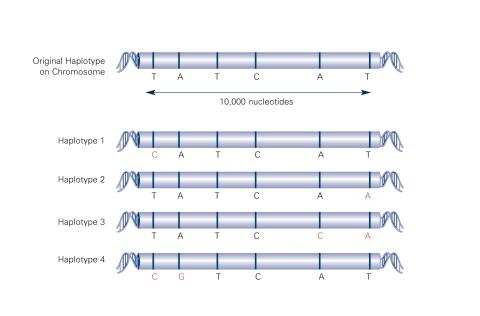
2567: Haplotypes (with labels)
2567: Haplotypes (with labels)
Haplotypes are combinations of gene variants that are likely to be inherited together within the same chromosomal region. In this example, an original haplotype (top) evolved over time to create three newer haplotypes that each differ by a few nucleotides (red). See image 2566 for an unlabeled version of this illustration. Featured in The New Genetics.
Crabtree + Company
View Media
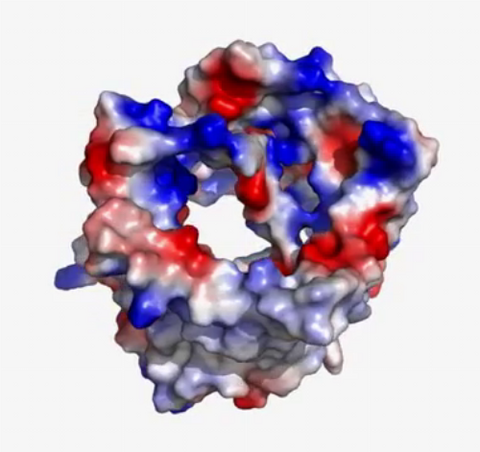
2571: VDAC video 02
2571: VDAC video 02
This video shows the structure of the pore-forming protein VDAC-1 from humans. This molecule mediates the flow of products needed for metabolism--in particular the export of ATP--across the outer membrane of mitochondria, the power plants for eukaryotic cells. VDAC-1 is involved in metabolism and the self-destruction of cells--two biological processes central to health.
Related to videos 2570 and 2572.
Related to videos 2570 and 2572.
Gerhard Wagner, Harvard Medical School
View Media
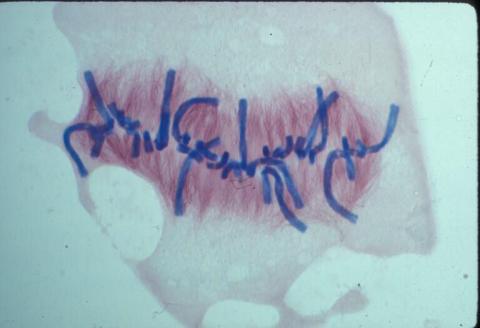
1021: Lily mitosis 08
1021: Lily mitosis 08
A light microscope image of a cell from the endosperm of an African globe lily (Scadoxus katherinae). This is one frame of a time-lapse sequence that shows cell division in action. The lily is considered a good organism for studying cell division because its chromosomes are much thicker and easier to see than human ones. Staining shows microtubules in red and chromosomes in blue. Here, condensed chromosomes are clearly visible and lined up.
Related to images 1010, 1011, 1012, 1013, 1014, 1015, 1016, 1017, 1018, and 1019.
Related to images 1010, 1011, 1012, 1013, 1014, 1015, 1016, 1017, 1018, and 1019.
Andrew S. Bajer, University of Oregon, Eugene
View Media
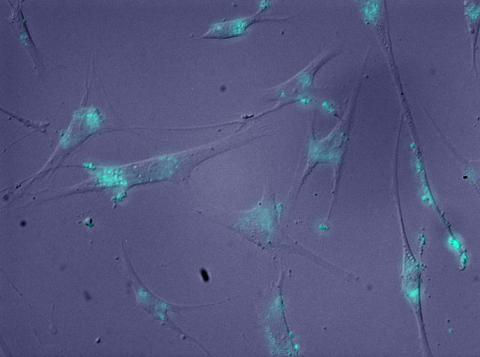
2600: Molecules blocking Huntington's protein production
2600: Molecules blocking Huntington's protein production
The molecules that glow blue in these cultured cells prevent the expression of the mutant proteins that cause Huntington's disease. Biochemist David Corey and others at UT Southwestern Medical Center designed the molecules to specifically target the genetic repeats that code for harmful proteins in people with Huntington's disese. People with Huntington's disease and similar neurodegenerative disorders often have extra copies of a gene segment. Moving from cell cultures to animals will help researchers further explore the potential of their specially crafted molecule to treat brain disorders. In addition to NIGMS, NIH's National Institute of Neurological Disorders and Stroke and National Institute of Biomedical Imaging and Bioengineering also funded this work.
Jiaxin Hu, David W. Dodd and Robert H. E. Hudson, UT Southwestern Medical Center
View Media
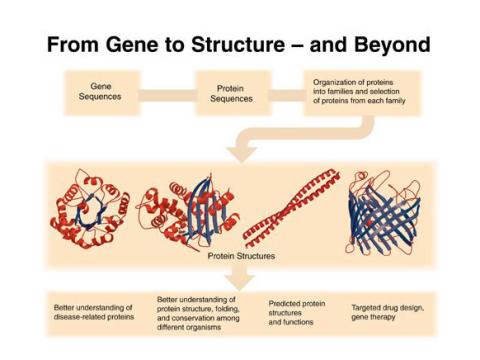
2363: PSI: from genes to structures
2363: PSI: from genes to structures
The goal of the Protein Structure Initiative (PSI) is to determine the three-dimensional shapes of a wide range of proteins by solving the structures of representative members of each protein family found in nature. The collection of structures should serve as a valuable resource for biomedical research scientists.
National Institute of General Medical Sciences
View Media
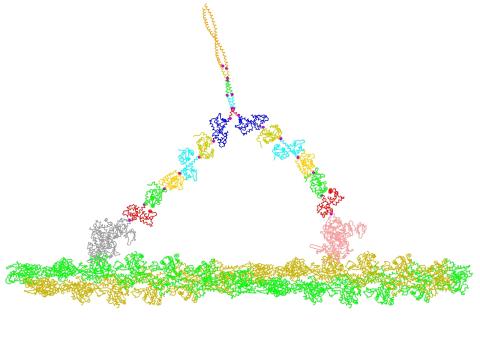
2754: Myosin V binding to actin
2754: Myosin V binding to actin
This simulation of myosin V binding to actin was created using the software tool Protein Mechanica. With Protein Mechanica, researchers can construct models using information from a variety of sources: crystallography, cryo-EM, secondary structure descriptions, as well as user-defined solid shapes, such as spheres and cylinders. The goal is to enable experimentalists to quickly and easily simulate how different parts of a molecule interact.
Simbios, NIH Center for Biomedical Computation at Stanford
View Media