Switch to List View
Image and Video Gallery
This is a searchable collection of scientific photos, illustrations, and videos. The images and videos in this gallery are licensed under Creative Commons Attribution Non-Commercial ShareAlike 3.0. This license lets you remix, tweak, and build upon this work non-commercially, as long as you credit and license your new creations under identical terms.
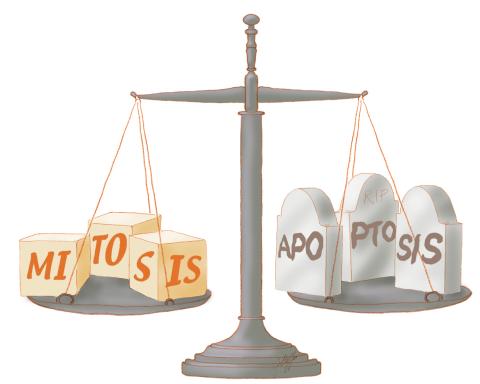
1336: Life in balance
1336: Life in balance
Mitosis creates cells, and apoptosis kills them. The processes often work together to keep us healthy.
Judith Stoffer
View Media
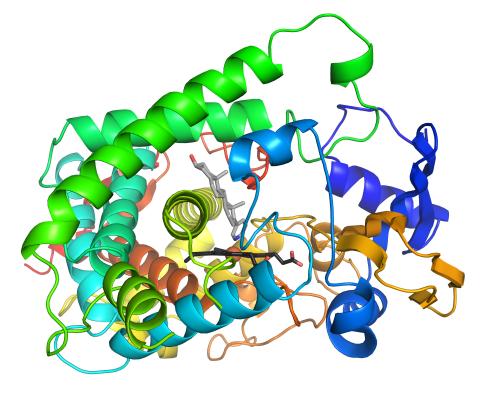
3326: Cytochrome structure with anticancer drug
3326: Cytochrome structure with anticancer drug
This image shows the structure of the CYP17A1 enzyme (ribbons colored from blue N-terminus to red C-terminus), with the associated heme colored black. The prostate cancer drug abiraterone is colored gray. Cytochrome P450 enzymes bind to and metabolize a variety of chemicals, including drugs. Cytochrome P450 17A1 also helps create steroid hormones. Emily Scott's lab is studying how CYP17A1 could be selectively inhibited to treat prostate cancer. She and graduate student Natasha DeVore elucidated the structure shown using X-ray crystallography. Dr. Scott created the image (both white bg and transparent bg) for the NIGMS image gallery. See the "Medium-Resolution Image" for a PNG version of the image that is transparent.
Emily Scott, University of Kansas
View Media
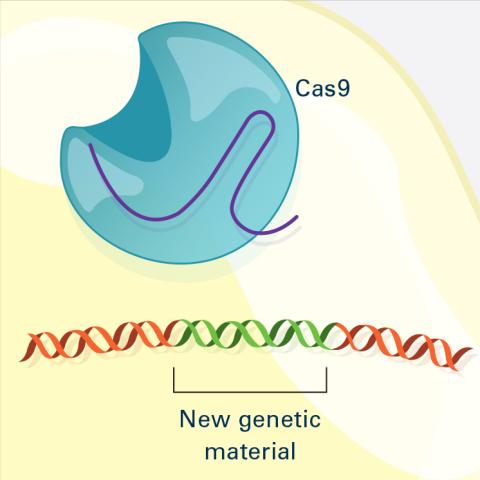
6488: CRISPR Illustration Frame 4
6488: CRISPR Illustration Frame 4
This illustration shows, in simplified terms, how the CRISPR-Cas9 system can be used as a gene-editing tool. The CRISPR system has two components joined together: a finely tuned targeting device (a small strand of RNA programmed to look for a specific DNA sequence) and a strong cutting device (an enzyme called Cas9 that can cut through a double strand of DNA). This frame (4 out of 4) shows a repaired DNA strand with new genetic material that researchers can introduce, which the cell automatically incorporates into the gap when it repairs the broken DNA.
For an explanation and overview of the CRISPR-Cas9 system, see the iBiology video, and find the full CRIPSR illustration here.
For an explanation and overview of the CRISPR-Cas9 system, see the iBiology video, and find the full CRIPSR illustration here.
National Institute of General Medical Sciences.
View Media
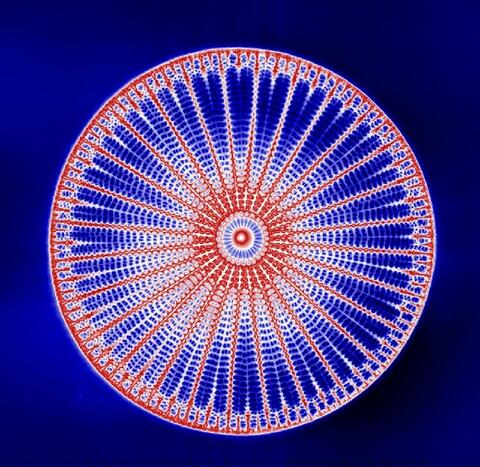
6902: Arachnoidiscus diatom
6902: Arachnoidiscus diatom
An Arachnoidiscus diatom with a diameter of 190µm. Diatoms are microscopic algae that have cell walls made of silica, which is the strongest known biological material relative to its density. In Arachnoidiscus, the cell wall is a radially symmetric pillbox-like shell composed of overlapping halves that contain intricate and delicate patterns. Sometimes, Arachnoidiscus is called “a wheel of glass.”
This image was taken with the orientation-independent differential interference contrast microscope.
This image was taken with the orientation-independent differential interference contrast microscope.
Michael Shribak, Marine Biological Laboratory/University of Chicago.
View Media
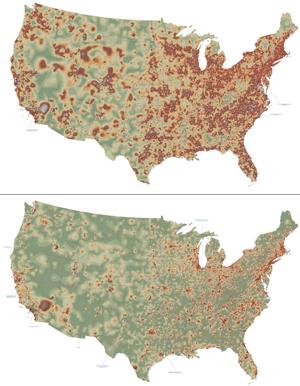
2320: Mapping disease spread
2320: Mapping disease spread
How far and fast an infectious disease spreads across a community depends on many factors, including transportation. These U.S. maps, developed as part of an international study to simulate and analyze disease spread, chart daily commuting patterns. They show where commuters live (top) and where they travel for work (bottom). Green represents the fewest number of people whereas orange, brown, and white depict the most. Such information enables researchers and policymakers to visualize how an outbreak in one area can spread quickly across a geographic region.
David Chrest, RTI International
View Media
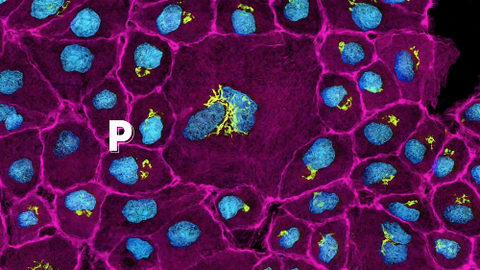
6538: Pathways: The Fascinating Cells of Research Organisms
6538: Pathways: The Fascinating Cells of Research Organisms
Learn how research organisms, such as fruit flies and mice, can help us understand and treat human diseases. Discover more resources from NIGMS’ Pathways collaboration with Scholastic. View the video on YouTube for closed captioning.
National Institute of General Medical Sciences
View Media
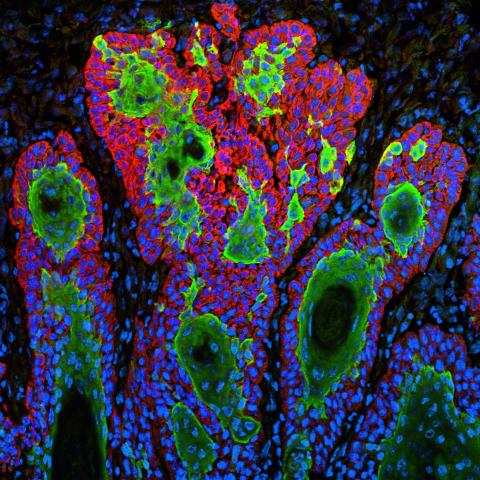
3628: Skin cancer cells (squamous cell carcinoma)
3628: Skin cancer cells (squamous cell carcinoma)
This image shows the uncontrolled growth of cells in squamous cell carcinoma, the second most common form of skin cancer. If caught early, squamous cell carcinoma is usually not life-threatening.
This image was part of the Life: Magnified exhibit that ran from June 3, 2014, to January 21, 2015, at Dulles International Airport.
This image was part of the Life: Magnified exhibit that ran from June 3, 2014, to January 21, 2015, at Dulles International Airport.
Markus Schober and Elaine Fuchs, The Rockefeller University
View Media
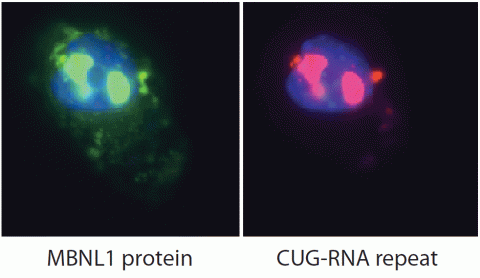
2727: Proteins related to myotonic dystrophy
2727: Proteins related to myotonic dystrophy
Myotonic dystrophy is thought to be caused by the binding of a protein called Mbnl1 to abnormal RNA repeats. In these two images of the same muscle precursor cell, the top image shows the location of the Mbnl1 splicing factor (green) and the bottom image shows the location of RNA repeats (red) inside the cell nucleus (blue). The white arrows point to two large foci in the cell nucleus where Mbnl1 is sequestered with RNA.
Manuel Ares, University of California, Santa Cruz
View Media
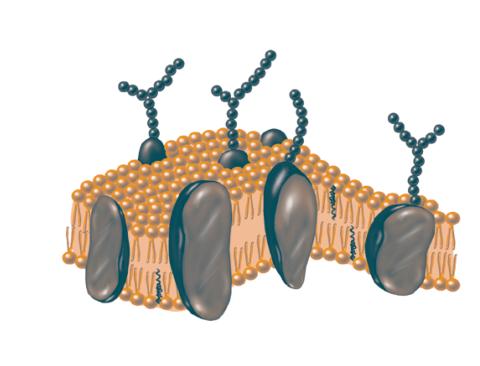
2523: Plasma membrane
2523: Plasma membrane
The plasma membrane is a cell's protective barrier. See image 2524 for a labeled version of this illustration. Featured in The Chemistry of Health.
Crabtree + Company
View Media
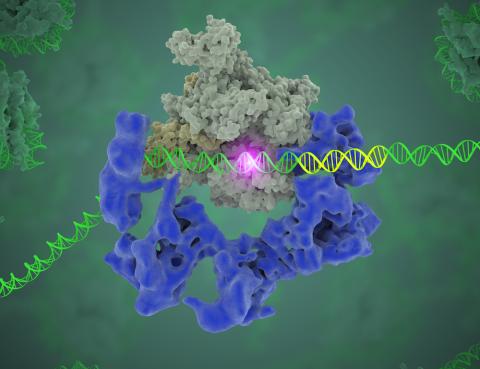
3766: TFIID complex binds DNA to start gene transcription
3766: TFIID complex binds DNA to start gene transcription
Gene transcription is a process by which the genetic information encoded in DNA is transcribed into RNA. It's essential for all life and requires the activity of proteins, called transcription factors, that detect where in a DNA strand transcription should start. In eukaryotes (i.e., those that have a nucleus and mitochondria), a protein complex comprising 14 different proteins is responsible for sniffing out transcription start sites and starting the process. This complex, called TFIID, represents the core machinery to which an enzyme, named RNA polymerase, can bind to and read the DNA and transcribe it to RNA. Scientists have used cryo-electron microscopy (cryo-EM) to visualize the TFIID-RNA polymerase-DNA complex in unprecedented detail. In this illustration, TFIID (blue) contacts the DNA and recruits the RNA polymerase (gray) for gene transcription. The start of the transcribed gene is shown with a flash of light. To learn more about the research that has shed new light on gene transcription, see this news release from Berkeley Lab. Related to video 5730.
Eva Nogales, Berkeley Lab
View Media
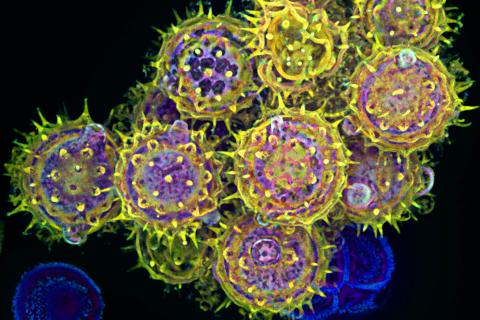
3609: Pollen grains: male germ cells in plants and a cause of seasonal allergies
3609: Pollen grains: male germ cells in plants and a cause of seasonal allergies
Those of us who get sneezy and itchy-eyed every spring or fall may have pollen grains, like those shown here, to blame. Pollen grains are the male germ cells of plants, released to fertilize the corresponding female plant parts. When they are instead inhaled into human nasal passages, they can trigger allergies.
This image was part of the Life: Magnified exhibit that ran from June 3, 2014, to January 21, 2015, at Dulles International Airport.
This image was part of the Life: Magnified exhibit that ran from June 3, 2014, to January 21, 2015, at Dulles International Airport.
Edna, Gil, and Amit Cukierman, Fox Chase Cancer Center, Philadelphia, Pa.
View Media
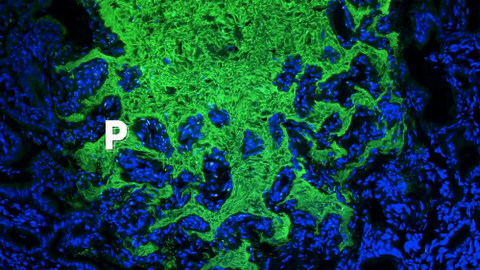
6539: Pathways: What is Basic Science?
6539: Pathways: What is Basic Science?
Learn about basic science, sometimes called “pure” or “fundamental” science, and how it contributes to the development of medical treatments. Discover more resources from NIGMS’ Pathways collaboration with Scholastic. View the video on YouTube for closed captioning.
National Institute of General Medical Sciences
View Media
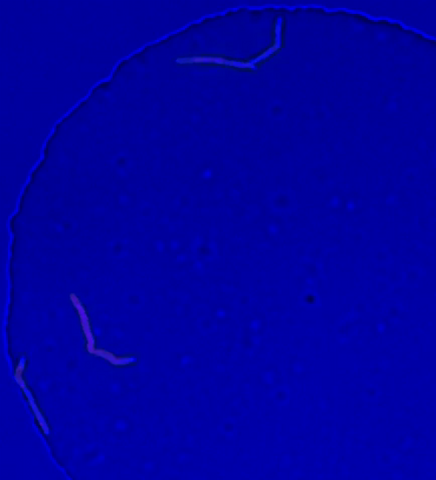
5752: Genetically identical mycobacteria respond differently to antibiotic 2
5752: Genetically identical mycobacteria respond differently to antibiotic 2
Antibiotic resistance in microbes is a serious health concern. So researchers have turned their attention to how bacteria undo the action of some antibiotics. Here, scientists set out to find the conditions that help individual bacterial cells survive in the presence of the antibiotic rifampicin. The research team used Mycobacterium smegmatis, a more harmless relative of Mycobacterium tuberculosis, which infects the lung and other organs to cause serious disease.
In this video, genetically identical mycobacteria are growing in a miniature growth chamber called a microfluidic chamber. Using live imaging, the researchers found that individual mycobacteria will respond differently to the antibiotic, depending on the growth stage and other timing factors. The researchers used genetic tagging with green fluorescent protein to distinguish cells that can resist rifampicin and those that cannot. With this gene tag, cells tolerant of the antibiotic light up in green and those that are susceptible in violet, enabling the team to monitor the cells' responses in real time.
To learn more about how the researchers studied antibiotic resistance in mycobacteria, see this news release from Tufts University. Related to image 5751.
In this video, genetically identical mycobacteria are growing in a miniature growth chamber called a microfluidic chamber. Using live imaging, the researchers found that individual mycobacteria will respond differently to the antibiotic, depending on the growth stage and other timing factors. The researchers used genetic tagging with green fluorescent protein to distinguish cells that can resist rifampicin and those that cannot. With this gene tag, cells tolerant of the antibiotic light up in green and those that are susceptible in violet, enabling the team to monitor the cells' responses in real time.
To learn more about how the researchers studied antibiotic resistance in mycobacteria, see this news release from Tufts University. Related to image 5751.
Bree Aldridge, Tufts University
View Media
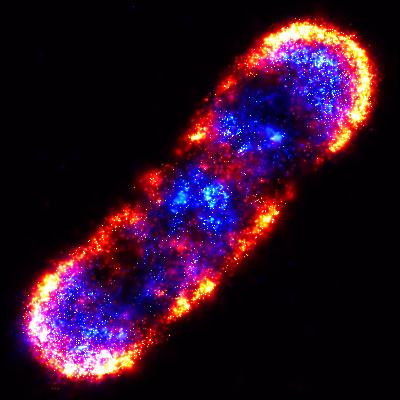
2771: Self-organizing proteins
2771: Self-organizing proteins
Under the microscope, an E. coli cell lights up like a fireball. Each bright dot marks a surface protein that tells the bacteria to move toward or away from nearby food and toxins. Using a new imaging technique, researchers can map the proteins one at a time and combine them into a single image. This lets them study patterns within and among protein clusters in bacterial cells, which don't have nuclei or organelles like plant and animal cells. Seeing how the proteins arrange themselves should help researchers better understand how cell signaling works.
View Media
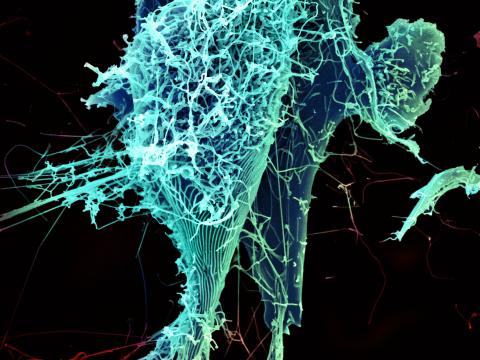
3619: String-like Ebola virus peeling off an infected cell
3619: String-like Ebola virus peeling off an infected cell
After multiplying inside a host cell, the stringlike Ebola virus is emerging to infect more cells. Ebola is a rare, often fatal disease that occurs primarily in tropical regions of sub-Saharan Africa. The virus is believed to spread to humans through contact with wild animals, especially fruit bats. It can be transmitted between one person and another through bodily fluids.
This image was part of the Life: Magnified exhibit that ran from June 3, 2014, to January 21, 2015, at Dulles International Airport.
This image was part of the Life: Magnified exhibit that ran from June 3, 2014, to January 21, 2015, at Dulles International Airport.
Heinz Feldmann, Peter Jahrling, Elizabeth Fischer and Anita Mora, National Institute of Allergy and Infectious Diseases, National Institutes of Health
View Media
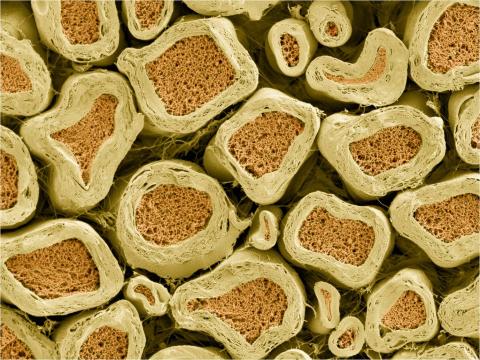
3397: Myelinated axons 2
3397: Myelinated axons 2
Top view of myelinated axons in a rat spinal root. Myelin is a type of fat that forms a sheath around and thus insulates the axon to protect it from losing the electrical current needed to transmit signals along the axon. The axoplasm inside the axon is shown in pink. Related to 3396.
Tom Deerinck, National Center for Microscopy and Imaging Research (NCMIR)
View Media
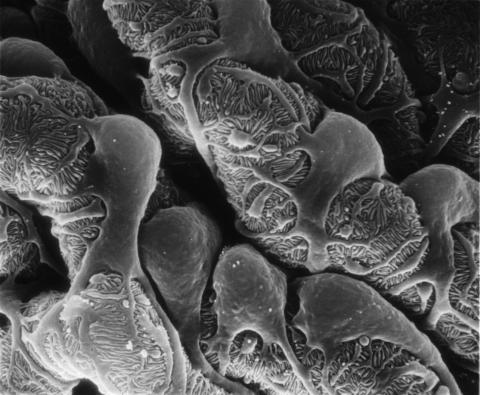
3565: Podocytes from a chronically diseased kidney
3565: Podocytes from a chronically diseased kidney
This scanning electron microscope (SEM) image shows podocytes--cells in the kidney that play a vital role in filtering waste from the bloodstream--from a patient with chronic kidney disease. This image first appeared in Princeton Journal Watch on October 4, 2013.
Olga Troyanskaya, Princeton University and Matthias Kretzler, University of Michigan
View Media
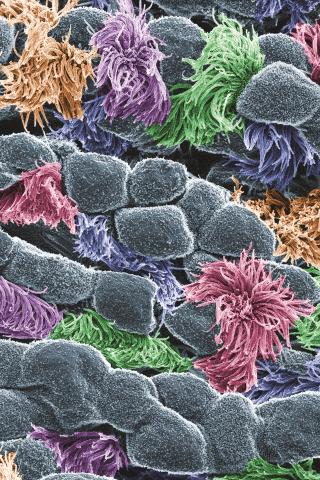
3646: Cells lining the trachea
3646: Cells lining the trachea
In this image, viewed with a ZEISS ORION NanoFab microscope, the community of cells lining a mouse airway is magnified more than 10,000 times. This collection of cells, known as the mucociliary escalator, is also found in humans. It is our first line of defense against inhaled bacteria, allergens, pollutants, and debris. Malfunctions in the system can cause or aggravate lung infections and conditions such as asthma and chronic obstructive pulmonary disease. The cells shown in gray secrete mucus, which traps inhaled particles. The colored cells sweep the mucus layer out of the lungs.
This image was part of the Life: Magnified exhibit that ran from June 3, 2014, to January 21, 2015, at Dulles International Airport.
This image was part of the Life: Magnified exhibit that ran from June 3, 2014, to January 21, 2015, at Dulles International Airport.
Eva Mutunga and Kate Klein, University of the District of Columbia and National Institute of Standards and Technology
View Media
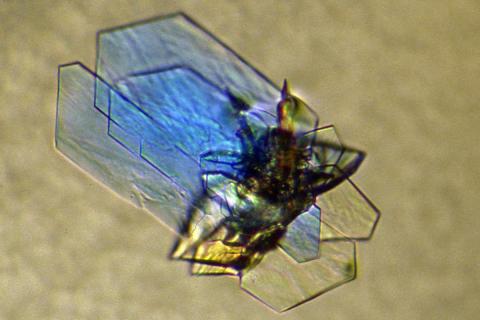
2410: DNase
2410: DNase
Crystals of DNase protein created for X-ray crystallography, which can reveal detailed, three-dimensional protein structures.
Alex McPherson, University of California, Irvine
View Media
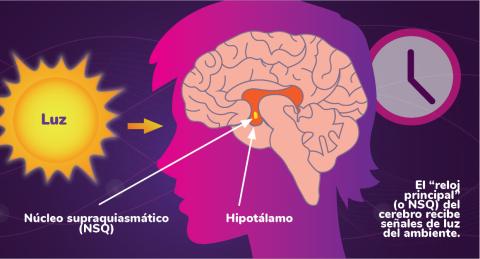
6614: Los ritmos circadianos y el núcleo supraquiasmático
6614: Los ritmos circadianos y el núcleo supraquiasmático
Los ritmos circadianos son cambios físicos, mentales y de comportamiento que siguen un ciclo de 24 horas. Los ritmos circadianos se ven influenciados por la luz y están regulados por el núcleo supraquiasmático del cerebro, a veces denominado el reloj principal.
Vea 6613 para la versión en inglés de esta infografía.
Vea 6613 para la versión en inglés de esta infografía.
NIGMS
View Media
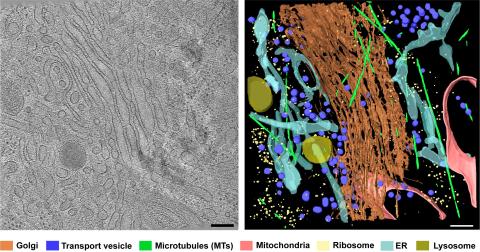
6606: Cryo-ET cross-section of the Golgi apparatus
6606: Cryo-ET cross-section of the Golgi apparatus
On the left, a cross-section slice of a rat pancreas cell captured using cryo-electron tomography (cryo-ET). On the right, a 3D, color-coded version of the image highlighting cell structures. Visible features include the folded sacs of the Golgi apparatus (copper), transport vesicles (medium-sized dark-blue circles), microtubules (neon green), ribosomes (small pale-yellow circles), and lysosomes (large yellowish-green circles). Black line (bottom right of the left image) represents 200 nm. This image is a still from video 6609.
Xianjun Zhang, University of Southern California.
View Media
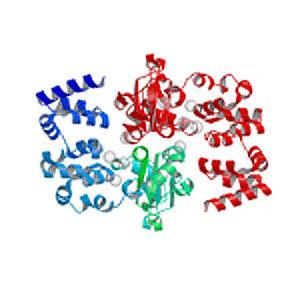
2345: Magnesium transporter protein from E. faecalis
2345: Magnesium transporter protein from E. faecalis
Structure of a magnesium transporter protein from an antibiotic-resistant bacterium (Enterococcus faecalis) found in the human gut. Featured as one of the June 2007 Protein Sructure Initiative Structures of the Month.
New York Structural GenomiX Consortium
View Media
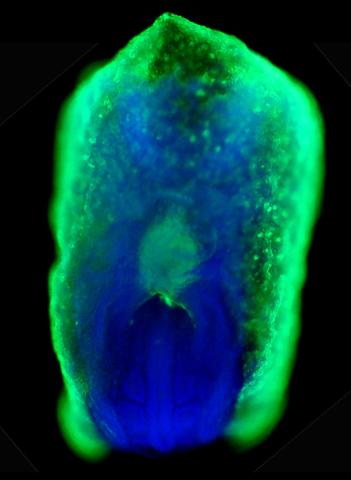
2607: Mouse embryo showing Smad4 protein
2607: Mouse embryo showing Smad4 protein
This eerily glowing blob isn't an alien or a creature from the deep sea--it's a mouse embryo just eight and a half days old. The green shell and core show a protein called Smad4. In the center, Smad4 is telling certain cells to begin forming the mouse's liver and pancreas. Researchers identified a trio of signaling pathways that help switch on Smad4-making genes, starting immature cells on the path to becoming organs. The research could help biologists learn how to grow human liver and pancreas tissue for research, drug testing and regenerative medicine. In addition to NIGMS, NIH's National Institute of Diabetes and Digestive and Kidney Diseases also supported this work.
Kenneth Zaret, Fox Chase Cancer Center
View Media
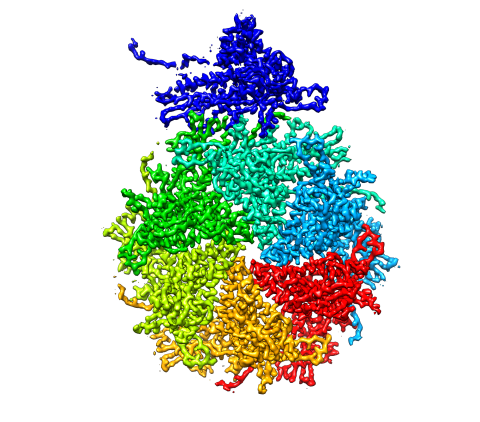
5875: Bacteriophage P22 capsid, detail
5875: Bacteriophage P22 capsid, detail
Detail of a subunit of the capsid, or outer cover, of bacteriophage P22, a virus that infects the Salmonella bacteria. Cryo-electron microscopy (cryo-EM) was used to capture details of the capsid proteins, each shown here in a separate color. Thousands of cryo-EM scans capture the structure and shape of all the individual proteins in the capsid and their position relative to other proteins. A computer model combines these scans into the image shown here. Related to image 5874.
Dr. Wah Chiu, Baylor College of Medicine
View Media

2762: Nucleolinus
2762: Nucleolinus
The nucleolinus is a cellular compartment that has been a lonely bystander in scientific endeavors. Although it's found in a range of species, its function has been mysterious—mainly because the structure is hard to visualize. An August 2010 study showed that the nucleolinus is crucial for cell division. When researchers zapped the structure with a laser, an egg cell didn't complete division. When the oocyte was fertilized after laser microsurgery (bottom right), the resulting zygote didn't form vital cell division structures (blue and yellow).
Mary Anne Alliegro, Marine Biological Laboratory
View Media
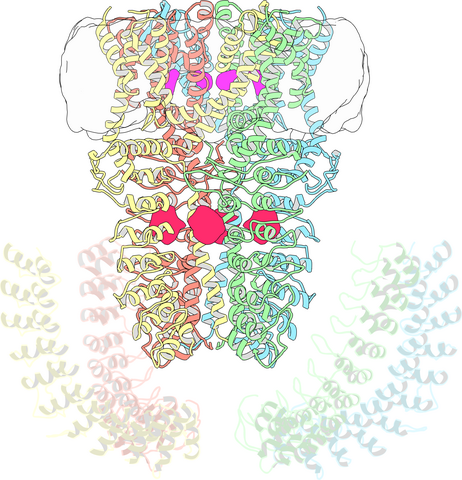
3747: Cryo-electron microscopy revealing the "wasabi receptor"
3747: Cryo-electron microscopy revealing the "wasabi receptor"
The TRPA1 protein is responsible for the burn you feel when you taste a bite of sushi topped with wasabi. Known therefore informally as the "wasabi receptor," this protein forms pores in the membranes of nerve cells that sense tastes or odors. Pungent chemicals like wasabi or mustard oil cause the pores to open, which then triggers a tingling or burn on our tongue. This receptor also produces feelings of pain in response to chemicals produced within our own bodies when our tissues are damaged or inflamed. Researchers used cryo-EM to reveal the structure of the wasabi receptor at a resolution of about 4 angstroms (a credit card is about 8 million angstroms thick). This detailed structure can help scientists understand both how we feel pain and how we can limit it by developing therapies to block the receptor. For more on cryo-EM see the blog post Cryo-Electron Microscopy Reveals Molecules in Ever Greater Detail.
Jean-Paul Armache, UCSF
View Media
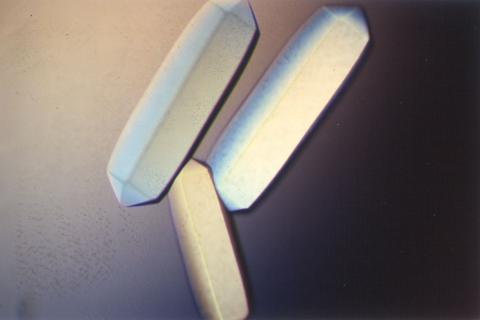
2411: Fungal lipase (2)
2411: Fungal lipase (2)
Crystals of fungal lipase protein created for X-ray crystallography, which can reveal detailed, three-dimensional protein structures.
Alex McPherson, University of California, Irvine
View Media
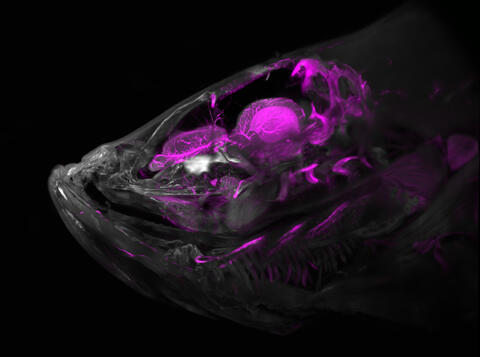
6934: Zebrafish head vasculature
6934: Zebrafish head vasculature
A zebrafish head with blood vessels shown in purple. Researchers often study zebrafish because they share many genes with humans, grow and reproduce quickly, and have see-through eggs and embryos, which make it easy to study early stages of development.
This image was captured using a light sheet microscope.
Related to video 6933.
This image was captured using a light sheet microscope.
Related to video 6933.
Prayag Murawala, MDI Biological Laboratory and Hannover Medical School.
View Media
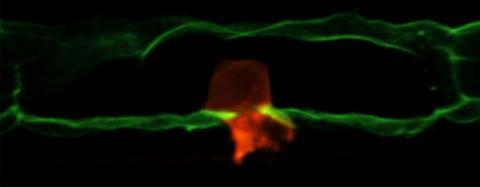
2707: Anchor cell in basement membrane
2707: Anchor cell in basement membrane
An anchor cell (red) pushes through the basement membrane (green) that surrounds it. Some cells are able to push through the tough basement barrier to carry out important tasks--and so can cancer cells, when they spread from one part of the body to another. No one has been able to recreate basement membranes in the lab and they're hard to study in humans, so Duke University researchers turned to the simple worm C. elegans. The researchers identified two molecules that help certain cells orient themselves toward and then punch through the worm's basement membrane. Studying these molecules and the genes that control them could deepen our understanding of cancer spread.
Elliott Hagedorn, Duke University.
View Media
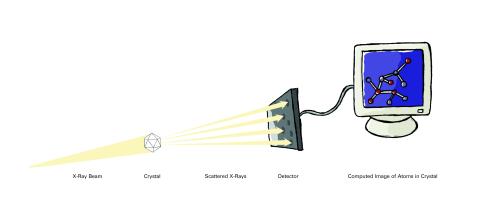
2512: X-ray crystallography (with labels)
2512: X-ray crystallography (with labels)
X-ray crystallography allows researchers to see structures too small to be seen by even the most powerful microscopes. To visualize the arrangement of atoms within molecules, researchers can use the diffraction patterns obtained by passing X-ray beams through crystals of the molecule. This is a common way for solving the structures of proteins. See image 2511 for an unlabeled version of this illustration. Featured in The Structures of Life.
Crabtree + Company
View Media
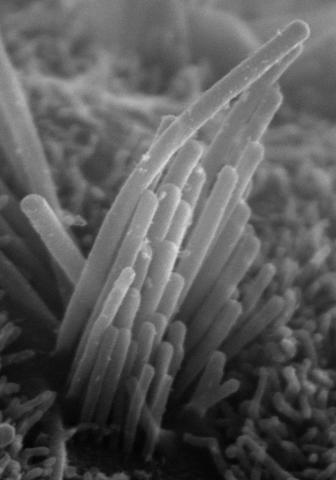
3272: Ear hair cells derived from embryonic stem cells
3272: Ear hair cells derived from embryonic stem cells
Mouse embryonic stem cells matured into this bundle of hair cells similar to the ones that transmit sound in the ear. These cells could one day be transplanted as a therapy for some forms of deafness, or they could be used to screen drugs to treat deafness. The hairs are shown at 23,000 times magnification via scanning electron microscopy. Image and caption information courtesy of the California Institute for Regenerative Medicine.
Stefen Heller, Stanford University, via CIRM
View Media
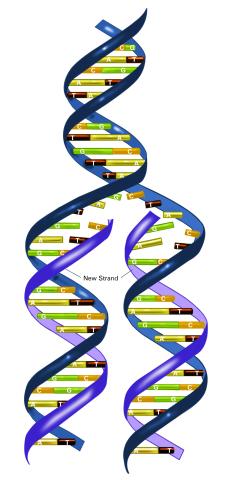
2544: DNA replication illustration (with labels)
2544: DNA replication illustration (with labels)
During DNA replication, each strand of the original molecule acts as a template for the synthesis of a new, complementary DNA strand. See image 2543 for an unlabeled version of this illustration. Featured in The New Genetics.
Crabtree + Company
View Media
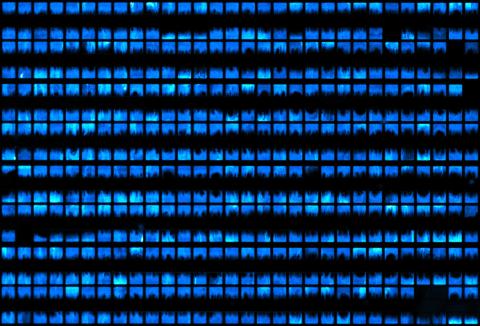
3266: Biopixels
3266: Biopixels
Bioengineers were able to coax bacteria to blink in unison on microfluidic chips. This image shows a small chip with about 500 blinking bacterial colonies or biopixels. Related to images 3265 and 3268. From a UC San Diego news release, "Researchers create living 'neon signs' composed of millions of glowing bacteria."
Jeff Hasty Lab, UC San Diego
View Media
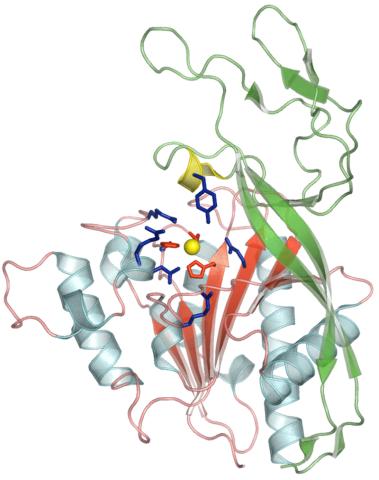
2352: Human aspartoacylase
2352: Human aspartoacylase
Model of aspartoacylase, a human enzyme involved in brain metabolism.
Center for Eukaryotic Structural Genomics, PSI
View Media
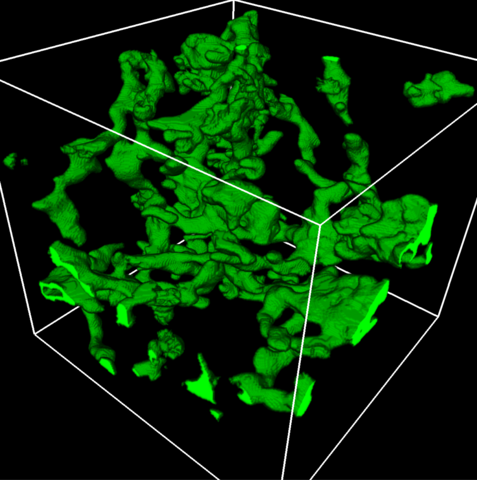
5857: 3D reconstruction of a tubular matrix in peripheral endoplasmic reticulum
5857: 3D reconstruction of a tubular matrix in peripheral endoplasmic reticulum
Detailed three-dimensional reconstruction of a tubular matrix in a thin section of the peripheral endoplasmic reticulum between the plasma membranes of the cell. The endoplasmic reticulum (ER) is a continuous membrane that extends like a net from the envelope of the nucleus outward to the cell membrane. The ER plays several roles within the cell, such as in protein and lipid synthesis and transport of materials between organelles. Shown here is a three-dimensional representation of the peripheral ER microtubules. Related to images 5855 and 5856
Jennifer Lippincott-Schwartz, Howard Hughes Medical Institute Janelia Research Campus, Virginia
View Media
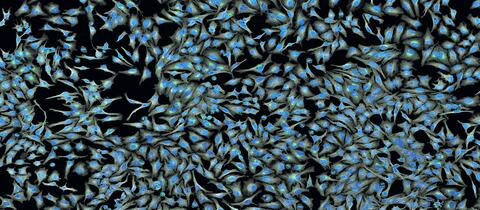
5761: A panorama view of cells
5761: A panorama view of cells
This photograph shows a panoramic view of HeLa cells, a cell line many researchers use to study a large variety of important research questions. The cells' nuclei containing the DNA are stained in blue and the cells' cytoskeletons in gray.
Tom Deerinck, National Center for Microscopy and Imaging Research
View Media
2431: Fruit fly embryo
2431: Fruit fly embryo
Cells in an early-stage fruit fly embryo, showing the DIAP1 protein (pink), an inhibitor of apoptosis.
Hermann Steller, Rockefeller University
View Media
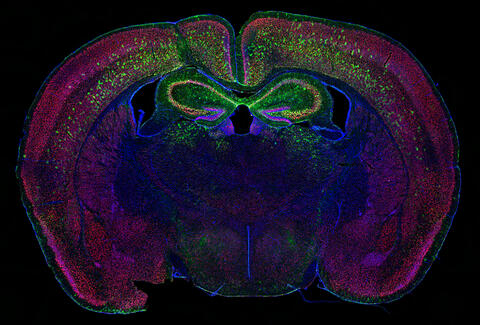
6780: Calling Cards in a mouse brain
6780: Calling Cards in a mouse brain
The green spots in this mouse brain are cells labeled with Calling Cards, a technology that records molecular events in brain cells as they mature. Understanding these processes during healthy development can guide further research into what goes wrong in cases of neuropsychiatric disorders. Also fluorescently labeled in this image are neurons (red) and nuclei (blue). Calling Cards and its application are described in the Cell paper “Self-Reporting Transposons Enable Simultaneous Readout of Gene Expression and Transcription Factor Binding in Single Cells” by Moudgil et al.; and the Proceedings of the National Academy of Sciences paper “A viral toolkit for recording transcription factor–DNA interactions in live mouse tissues” by Cammack et al. The technology was also featured in the NIH Director’s Blog post The Amazing Brain: Tracking Molecular Events with Calling Cards.
Related to video
Related to video
Allen Yen, Lab of Joseph Dougherty, Washington University School of Medicine in St. Louis.
View Media
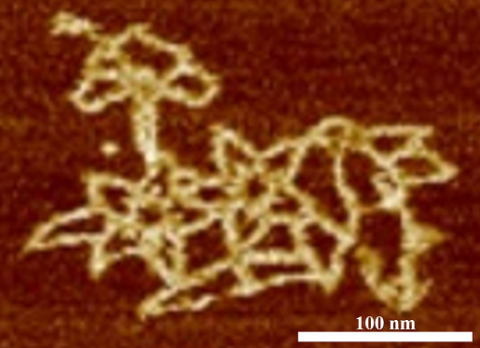
3690: Microscopy image of bird-and-flower DNA origami
3690: Microscopy image of bird-and-flower DNA origami
An atomic force microscopy image shows DNA folded into an intricate, computer-designed structure. Image is featured on Biomedical Beat blog post Cool Image: DNA Origami. See also related image 3689 .
Hao Yan, Arizona State University
View Media
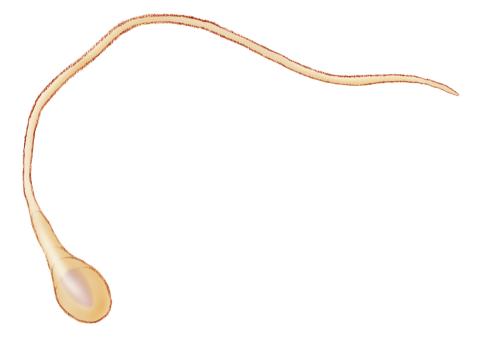
1293: Sperm cell
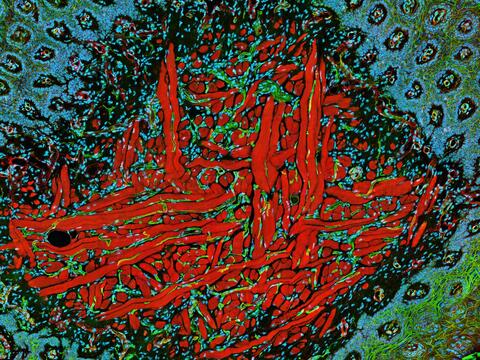
5811: NCMIR Tongue 2
5811: NCMIR Tongue 2
Microscopy image of a tongue. One in a series of two, see image 5810
National Center for Microscopy and Imaging Research (NCMIR)
View Media
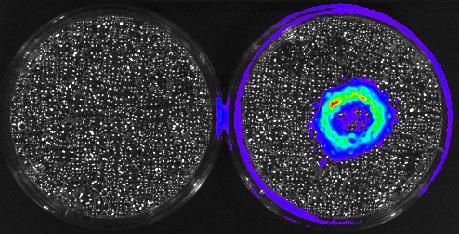
3480: Cancer Cells Glowing from Luciferin
3480: Cancer Cells Glowing from Luciferin
The activator cancer cell culture, right, contains a chemical that causes the cells to emit light when in the presence of immune cells.
Mark Sellmyer, Stanford University School of Medicine
View Media
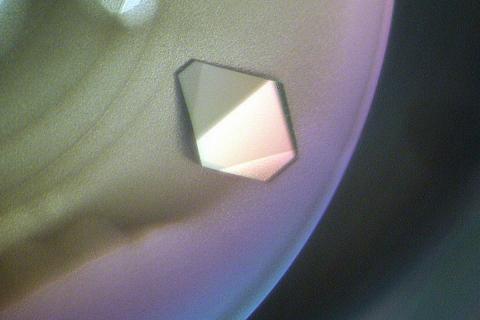
2409: Bacterial glucose isomerase
2409: Bacterial glucose isomerase
A crystal of bacterial glucose isomerase protein created for X-ray crystallography, which can reveal detailed, three-dimensional protein structures.
Alex McPherson, University of California, Irvine
View Media
1082: Natcher Building 02
1082: Natcher Building 02
NIGMS staff are located in the Natcher Building on the NIH campus.
Alisa Machalek, National Institute of General Medical Sciences
View Media
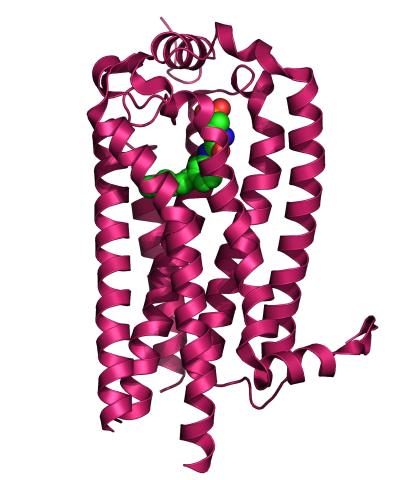
3362: Sphingolipid S1P1 receptor
3362: Sphingolipid S1P1 receptor
The receptor is shown bound to an antagonist, ML056.
Raymond Stevens, The Scripps Research Institute
View Media
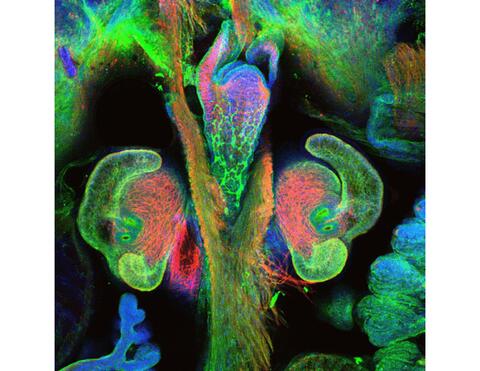
7017: The nascent juvenile light organ of the Hawaiian bobtail squid
7017: The nascent juvenile light organ of the Hawaiian bobtail squid
A light organ (~0.5 mm across) of a Hawaiian bobtail squid, Euprymna scolopes, with different tissues are stained various colors. The two pairs of ciliated appendages, or “arms,” on the sides of the organ move Vibrio fischeri bacterial cells closer to the two sets of three pores (two seen in this image) at the base of the arms that each lead to an interior crypt. This image was taken using a confocal fluorescence microscope.
Related to images 7016, 7018, 7019, and 7020.
Related to images 7016, 7018, 7019, and 7020.
Margaret J. McFall-Ngai, Carnegie Institution for Science/California Institute of Technology, and Edward G. Ruby, California Institute of Technology.
View Media
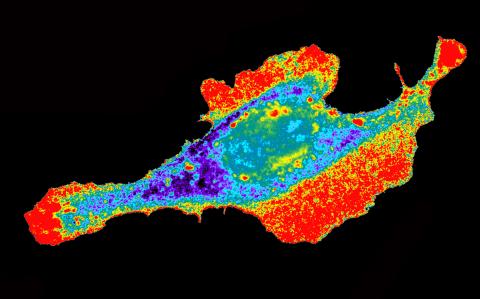
2453: Seeing signaling protein activation in cells 03
2453: Seeing signaling protein activation in cells 03
Cdc42, a member of the Rho family of small guanosine triphosphatase (GTPase) proteins, regulates multiple cell functions, including motility, proliferation, apoptosis, and cell morphology. In order to fulfill these diverse roles, the timing and location of Cdc42 activation must be tightly controlled. Klaus Hahn and his research group use special dyes designed to report protein conformational changes and interactions, here in living neutrophil cells. Warmer colors in this image indicate higher levels of activation. Cdc42 looks to be activated at cell protrusions.
Related to images 2451, 2452, and 2454.
Related to images 2451, 2452, and 2454.
Klaus Hahn, University of North Carolina, Chapel Hill Medical School
View Media
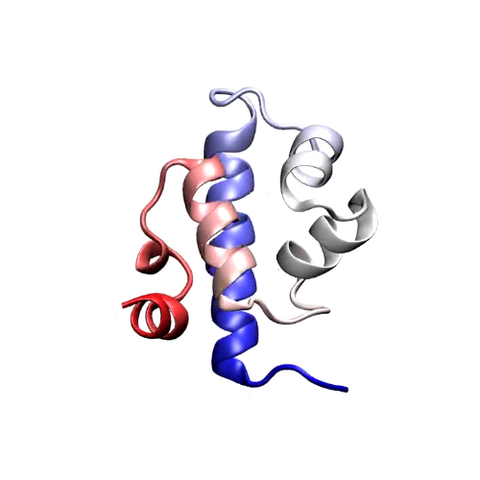
3391: Protein folding video
3391: Protein folding video
Proteins are long chains of amino acids. Each protein has a unique amino acid sequence. It is still a mystery how a protein folds into the proper shape based on its sequence. Scientists hope that one day they can "watch" this folding process for any given protein. The dream has been realized, at least partially, through the use of computer simulation.
Theoretical and Computational Biophysics Group
View Media
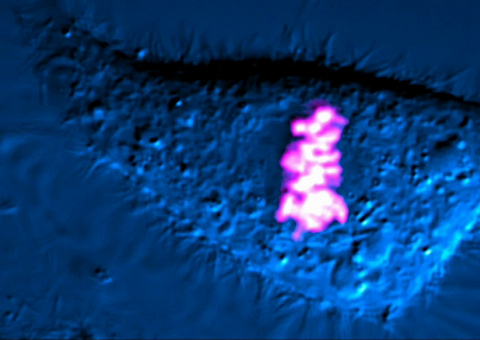
6965: Dividing cell
6965: Dividing cell
As this cell was undergoing cell division, it was imaged with two microscopy techniques: differential interference contrast (DIC) and confocal. The DIC view appears in blue and shows the entire cell. The confocal view appears in pink and shows the chromosomes.
Dylan T. Burnette, Vanderbilt University School of Medicine.
View Media
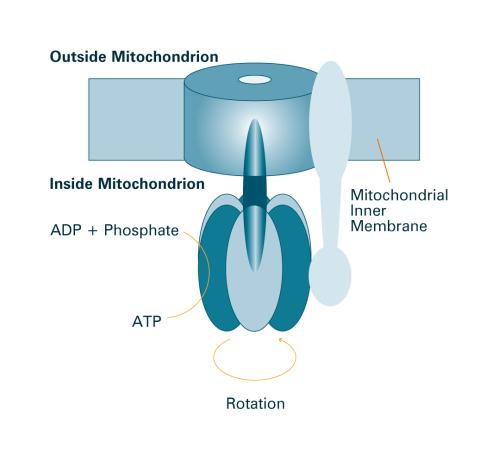
2518: ATP synthase (with labels)
2518: ATP synthase (with labels)
The world's smallest motor, ATP synthase, generates energy for the cell. See image 2517 for an unlabeled version of this illustration. Featured in The Chemistry of Health.
Crabtree + Company
View Media