Switch to List View
Image and Video Gallery
This is a searchable collection of scientific photos, illustrations, and videos. The images and videos in this gallery are licensed under Creative Commons Attribution Non-Commercial ShareAlike 3.0. This license lets you remix, tweak, and build upon this work non-commercially, as long as you credit and license your new creations under identical terms.
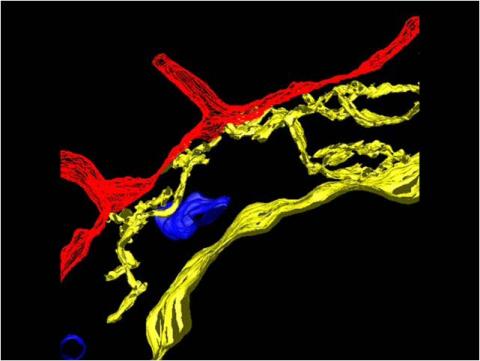
2636: Computer model of cell membrane
2636: Computer model of cell membrane
A computer model of the cell membrane, where the plasma membrane is red, endoplasmic reticulum is yellow, and mitochondria are blue. This image relates to a July 27, 2009 article in Computing Life.
Bridget Wilson, University of New Mexico
View Media
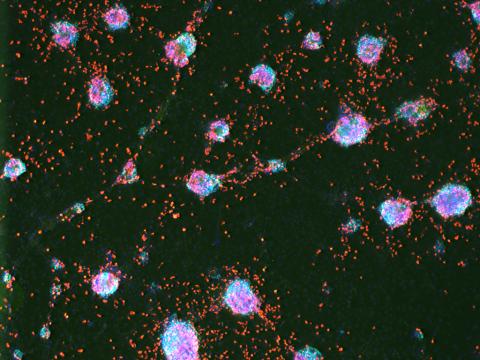
3277: Human ES cells turn into insulin-producing cells
3277: Human ES cells turn into insulin-producing cells
Human embryonic stem cells were differentiated into cells like those found in the pancreas (blue), which give rise to insulin-producing cells (red). When implanted in mice, the stem cell-derived pancreatic cells can replace the insulin that isn't produced in type 1 diabetes. Image and caption information courtesy of the California Institute for Regenerative Medicine.
Eugene Brandon, ViaCyte, via CIRM
View Media
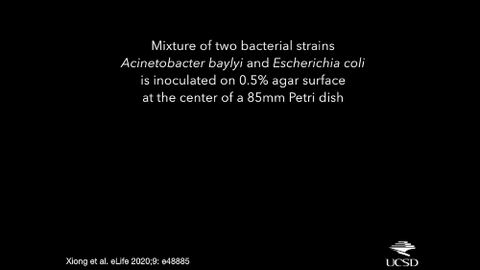
6550: Time-lapse video of floral pattern in a mixture of two bacterial species, Acinetobacter baylyi and Escherichia coli, grown on a semi-solid agar for 24 hours
6550: Time-lapse video of floral pattern in a mixture of two bacterial species, Acinetobacter baylyi and Escherichia coli, grown on a semi-solid agar for 24 hours
This time-lapse video shows the emergence of a flower-like pattern in a mixture of two bacterial species, motile Acinetobacter baylyi and non-motile Escherichia coli (green), that are grown together for 24 hours on 0.75% agar surface from a small inoculum in the center of a Petri dish.
See 6557 for a photo of this process at 24 hours on 0.75% agar surface.
See 6553 for a photo of this process at 48 hours on 1% agar surface.
See 6555 for another photo of this process at 48 hours on 1% agar surface.
See 6556 for a photo of this process at 72 hours on 0.5% agar surface.
See 6557 for a photo of this process at 24 hours on 0.75% agar surface.
See 6553 for a photo of this process at 48 hours on 1% agar surface.
See 6555 for another photo of this process at 48 hours on 1% agar surface.
See 6556 for a photo of this process at 72 hours on 0.5% agar surface.
L. Xiong et al, eLife 2020;9: e48885
View Media
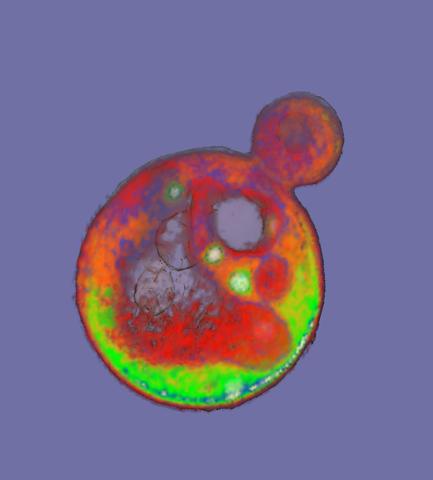
2307: Cells frozen in time
2307: Cells frozen in time
The fledgling field of X-ray microscopy lets researchers look inside whole cells rapidly frozen to capture their actions at that very moment. Here, a yeast cell buds before dividing into two. Colors show different parts of the cell. Seeing whole cells frozen in time will help scientists observe cells' complex structures and follow how molecules move inside them.
Carolyn Larabell, University of California, San Francisco, and the Lawrence Berkeley National Laboratory
View Media
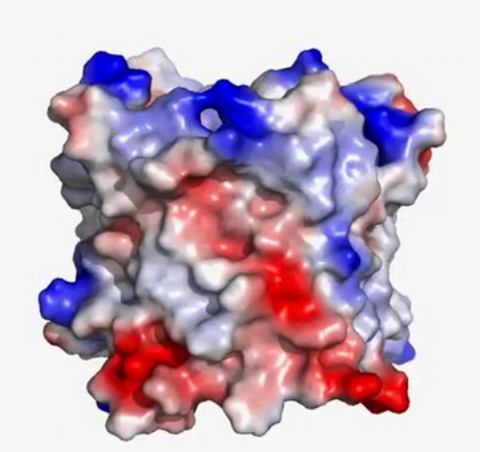
2572: VDAC video 03
2572: VDAC video 03
This video shows the structure of the pore-forming protein VDAC-1 from humans. This molecule mediates the flow of products needed for metabolism--in particular the export of ATP--across the outer membrane of mitochondria, the power plants for eukaryotic cells. VDAC-1 is involved in metabolism and the self-destruction of cells--two biological processes central to health.
Related to videos 2570 and 2571.
Related to videos 2570 and 2571.
Gerhard Wagner, Harvard Medical School
View Media
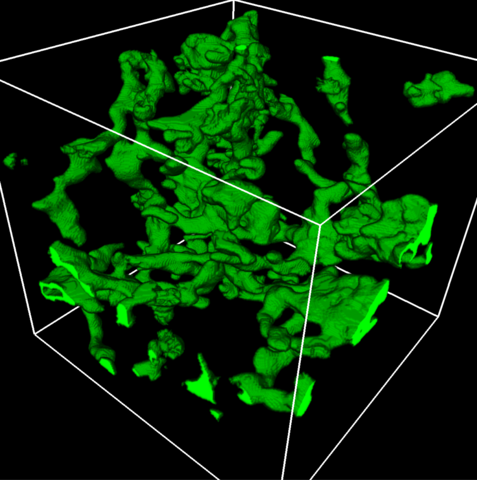
5857: 3D reconstruction of a tubular matrix in peripheral endoplasmic reticulum
5857: 3D reconstruction of a tubular matrix in peripheral endoplasmic reticulum
Detailed three-dimensional reconstruction of a tubular matrix in a thin section of the peripheral endoplasmic reticulum between the plasma membranes of the cell. The endoplasmic reticulum (ER) is a continuous membrane that extends like a net from the envelope of the nucleus outward to the cell membrane. The ER plays several roles within the cell, such as in protein and lipid synthesis and transport of materials between organelles. Shown here is a three-dimensional representation of the peripheral ER microtubules. Related to images 5855 and 5856
Jennifer Lippincott-Schwartz, Howard Hughes Medical Institute Janelia Research Campus, Virginia
View Media
2763: Fused, dicentric chromosomes
2763: Fused, dicentric chromosomes
This fused chromosome has two functional centromeres, shown as two sets of red and green dots. Centromeres are DNA/protein complexes that are key to splitting the chromosomes evenly during cell division. When dicentric chromosomes like this one are formed in a person, fertility problems or other difficulties may arise. Normal chromosomes carrying a single centromere (one set of red and green dots) are also visible in this image.
Beth A. Sullivan, Duke University
View Media
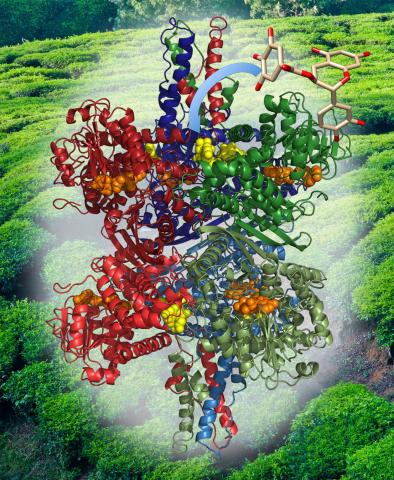
3421: Structure of Glutamate Dehydrogenase
3421: Structure of Glutamate Dehydrogenase
Some children are born with a mutation in a regulatory site on this enzyme that causes them to over-secrete insulin when they consume protein. We found that a compound from green tea (shown in the stick figure and by the yellow spheres on the enzyme) is able to block this hyperactivity when given to animals with this disorder.
Judy Coyle, Donald Danforth Plant Science Center
View Media
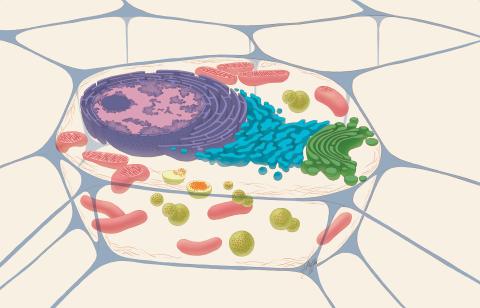
1274: Animal cell
1274: Animal cell
A typical animal cell, sliced open to reveal a cross-section of organelles.
Judith Stoffer
View Media
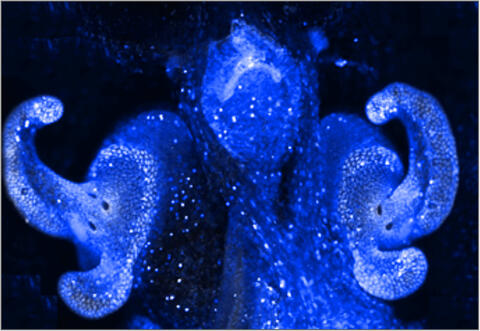
7016: Pores on the surface of the Hawaiian bobtail squid light organ
7016: Pores on the surface of the Hawaiian bobtail squid light organ
The light organ (~0.5 mm across) of a juvenile Hawaiian bobtail squid, Euprymna scolopes, stained blue. The two pairs of ciliated appendages, or “arms,” on the sides of the organ move Vibrio fischeri bacterial cells closer to the two sets of three pores at the base of the arms that each lead to an interior crypt. This image was taken using a confocal fluorescence microscope.
Related to images 7017, 7018, 7019, and 7020.
Related to images 7017, 7018, 7019, and 7020.
Margaret J. McFall-Ngai, Carnegie Institution for Science/California Institute of Technology, and Edward G. Ruby, California Institute of Technology.
View Media
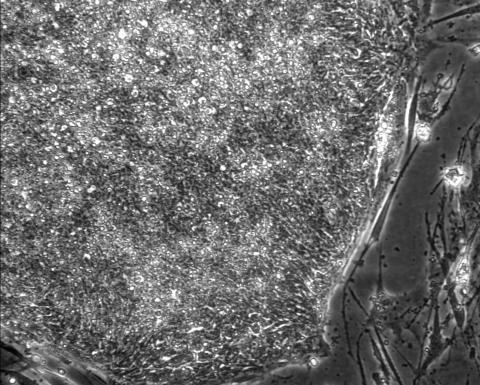
2603: Induced stem cells from adult skin 01
2603: Induced stem cells from adult skin 01
These cells are induced stem cells made from human adult skin cells that were genetically reprogrammed to mimic embryonic stem cells. The induced stem cells were made potentially safer by removing the introduced genes and the viral vector used to ferry genes into the cells, a loop of DNA called a plasmid. The work was accomplished by geneticist Junying Yu in the laboratory of James Thomson, a University of Wisconsin-Madison School of Medicine and Public Health professor and the director of regenerative biology for the Morgridge Institute for Research.
James Thomson, University of Wisconsin-Madison
View Media
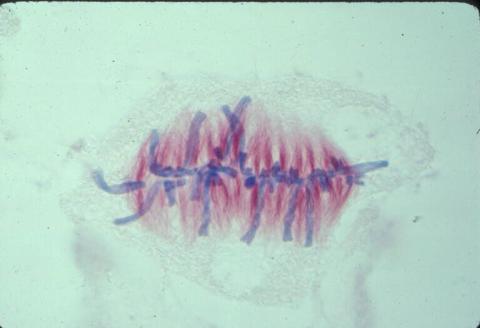
1017: Lily mitosis 07
1017: Lily mitosis 07
A light microscope image of a cell from the endosperm of an African globe lily (Scadoxus katherinae). This is one frame of a time-lapse sequence that shows cell division in action. The lily is considered a good organism for studying cell division because its chromosomes are much thicker and easier to see than human ones. Staining shows microtubules in red and chromosomes in blue. Here, condensed chromosomes are clearly visible and have lined up in the middle of the dividing cell.
Related to images 1010, 1011, 1012, 1013, 1014, 1015, 1016, 1018, 1019, and 1021.
Related to images 1010, 1011, 1012, 1013, 1014, 1015, 1016, 1018, 1019, and 1021.
Andrew S. Bajer, University of Oregon, Eugene
View Media
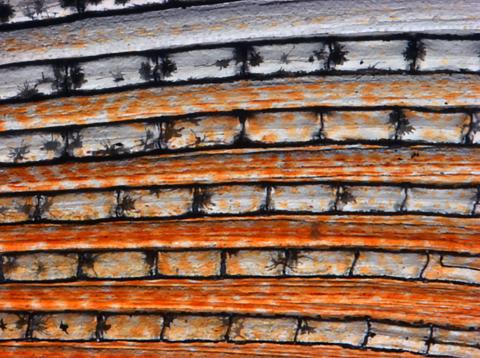
5757: Pigment cells in the fin of pearl danio
5757: Pigment cells in the fin of pearl danio
Pigment cells are cells that give skin its color. In fishes and amphibians, like frogs and salamanders, pigment cells are responsible for the characteristic skin patterns that help these organisms to blend into their surroundings or attract mates. The pigment cells are derived from neural crest cells, which are cells originating from the neural tube in the early embryo. This image shows pigment cells in the fin of pearl danio, a close relative of the popular laboratory animal zebrafish. Investigating pigment cell formation and migration in animals helps answer important fundamental questions about the factors that control pigmentation in the skin of animals, including humans. Related to images 5754, 5755, 5756 and 5758.
David Parichy, University of Washington
View Media
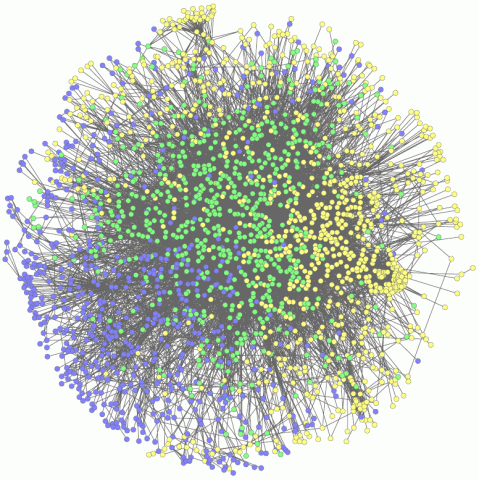
2735: Network Map
2735: Network Map
This network map shows the overlap (green) between the long QT syndrome (yellow) and epilepsy (blue) protein-interaction neighborhoods located within the human interactome. Researchers have learned to integrate genetic, cellular and clinical information to find out why certain medicines can trigger fatal heart arrhythmias. Featured in Computing Life magazine.
Seth Berger, Mount Sinai School of Medicine
View Media
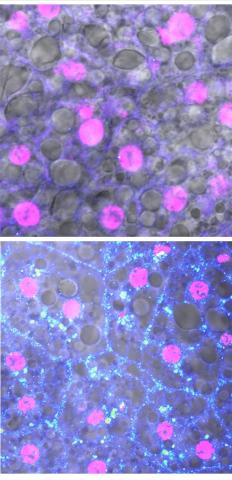
6984: Fruit fly starvation leads to adipokine accumulation
6984: Fruit fly starvation leads to adipokine accumulation
Adult Drosophila abdominal fat tissue showing cell nuclei labelled in magenta. The upper panel is from well-fed flies, and the lower panel is from flies that have been deprived of food for 4 hours. Starvation results in the accumulation of a key adipokine—a fat hormone (blue-green dots).
Related to images 6982, 6983, and 6985.
Related to images 6982, 6983, and 6985.
Akhila Rajan, Fred Hutchinson Cancer Center
View Media
2767: Research mentor and student
2767: Research mentor and student
A research mentor (Lori Eidson) and student (Nina Waldron, on the microscope) were 2009 members of the BRAIN (Behavioral Research Advancements In Neuroscience) program at Georgia State University in Atlanta. This program is an undergraduate summer research experience funded in part by NIGMS.
Elizabeth Weaver, Georgia State University
View Media
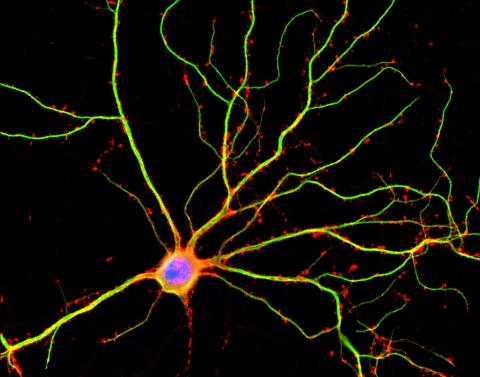
3687: Hippocampal neuron in culture
3687: Hippocampal neuron in culture
Hippocampal neuron in culture. Dendrites are green, dendritic spines are red and DNA in cell's nucleus is blue. Image is featured on Biomedical Beat blog post Anesthesia and Brain Cells: A Temporary Disruption?
Shelley Halpain, UC San Diego
View Media
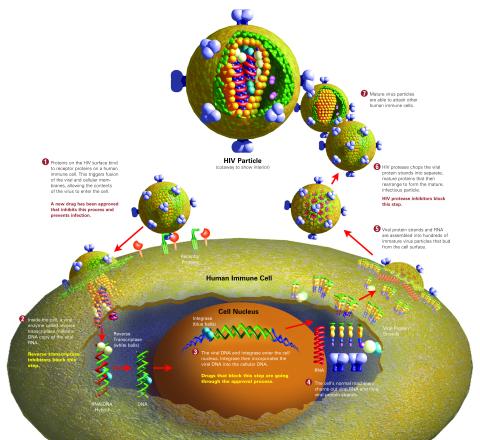
2515: Life of an AIDS virus (with labels and stages)
2515: Life of an AIDS virus (with labels and stages)
HIV is a retrovirus, a type of virus that carries its genetic material not as DNA but as RNA. Long before anyone had heard of HIV, researchers in labs all over the world studied retroviruses, tracing out their life cycle and identifying the key proteins the viruses use to infect cells. When HIV was identified as a retrovirus, these studies gave AIDS researchers an immediate jump-start. The previously identified viral proteins became initial drug targets. See images 2513 and 2514 for other versions of this illustration. Featured in The Structures of Life.
Crabtree + Company
View Media
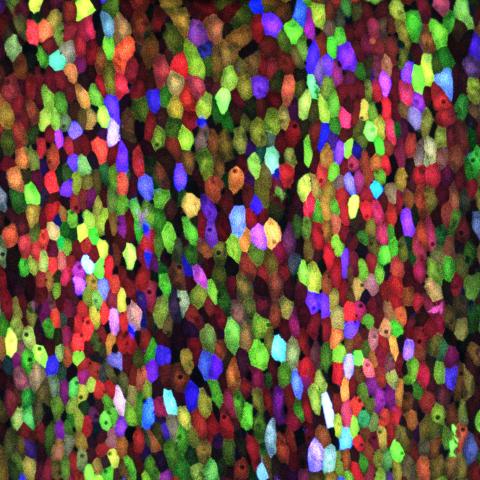
3782: A multicolored fish scale 1
3782: A multicolored fish scale 1
Each of the colored specs in this image is a cell on the surface of a fish scale. To better understand how wounds heal, scientists have inserted genes that make cells brightly glow in different colors into the skin cells of zebrafish, a fish often used in laboratory research. The colors enable the researchers to track each individual cell, for example, as it moves to the location of a cut or scrape over the course of several days. These technicolor fish endowed with glowing skin cells dubbed "skinbow" provide important insight into how tissues recover and regenerate after an injury.
For more information on skinbow fish, see the Biomedical Beat blog post Visualizing Skin Regeneration in Real Time and a press release from Duke University highlighting this research. Related to image 3783.
For more information on skinbow fish, see the Biomedical Beat blog post Visualizing Skin Regeneration in Real Time and a press release from Duke University highlighting this research. Related to image 3783.
Chen-Hui Chen and Kenneth Poss, Duke University
View Media
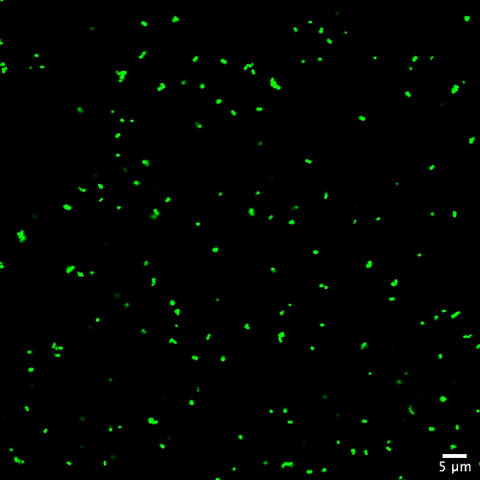
6805: Staphylococcus aureus aggregating upon contact with synovial fluid
6805: Staphylococcus aureus aggregating upon contact with synovial fluid
Staphylococcus aureus bacteria (green) grouping together upon contact with synovial fluid—a viscous substance found in joints. The formation of groups can help protect the bacteria from immune system defenses and from antibiotics, increasing the likelihood of an infection. This video is a 1-hour time lapse and was captured using a confocal laser scanning microscope.
More information about the research that produced this video can be found in the Journal of Bacteriology paper "In Vitro Staphylococcal Aggregate Morphology and Protection from Antibiotics Are Dependent on Distinct Mechanisms Arising from Postsurgical Joint Components and Fluid Motion" by Staats et al.
Related to images 6803 and 6804.
More information about the research that produced this video can be found in the Journal of Bacteriology paper "In Vitro Staphylococcal Aggregate Morphology and Protection from Antibiotics Are Dependent on Distinct Mechanisms Arising from Postsurgical Joint Components and Fluid Motion" by Staats et al.
Related to images 6803 and 6804.
Paul Stoodley, The Ohio State University.
View Media
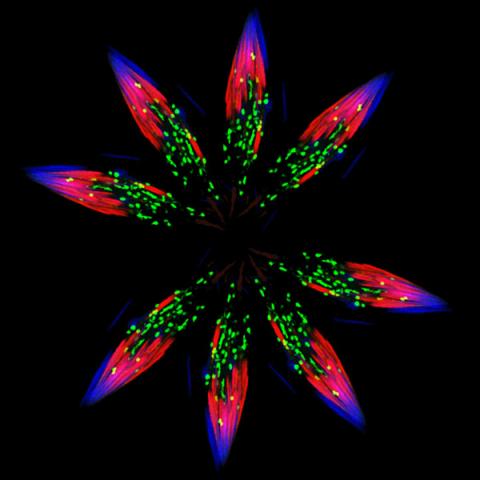
5888: Independence Day
5888: Independence Day
This graphic that resembles a firework was created from a picture of a fruit fly spermatid. This fruit fly spermatid recycles various molecules, including malformed or damaged proteins. Actin filaments (red) in the cell draw unwanted proteins toward a barrel-shaped structure called the proteasome (green clusters), which degrades the molecules into their basic parts for re-use.
Sigi Benjamin-Hong, Rockefeller University
View Media
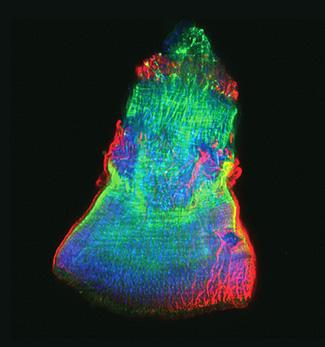
3593: Isolated Planarian Pharynx
3593: Isolated Planarian Pharynx
The feeding tube, or pharynx, of a planarian worm with cilia shown in red and muscle fibers shown in green
View Media
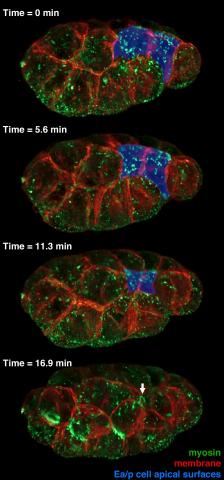
3297: Four timepoints in gastrulation
3297: Four timepoints in gastrulation
It has been said that gastrulation is the most important event in a person's life. This part of early embryonic development transforms a simple ball of cells and begins to define cell fate and the body axis. In a study published in Science magazine in March 2012, NIGMS grantee Bob Goldstein and his research group studied how contractions of actomyosin filaments in C. elegans and Drosophila embryos lead to dramatic rearrangements of cell and embryonic structure. This research is described in detail in the following article: "Triggering a Cell Shape Change by Exploiting Preexisting Actomyosin Contractions." In these images, myosin (green) and plasma membrane (red) are highlighted at four timepoints in gastrulation in the roundworm C. elegans. The blue highlights in the top three frames show how cells are internalized, and the site of closure around the involuting cells is marked with an arrow in the last frame. See related video 3334.
Bob Goldstein, University of North Carolina, Chapel Hill
View Media
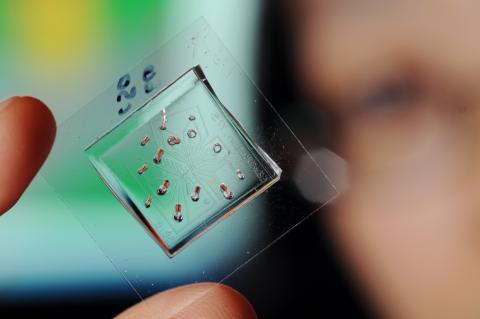
3475: Automated Worm Sorter - 4
3475: Automated Worm Sorter - 4
Georgia Tech associate professor Hang Lu holds a microfluidic chip that is part of a system that uses artificial intelligence and cutting-edge image processing to automatically examine large number of nematodes used for genetic research.
Georgia Tech/Gary Meek
View Media
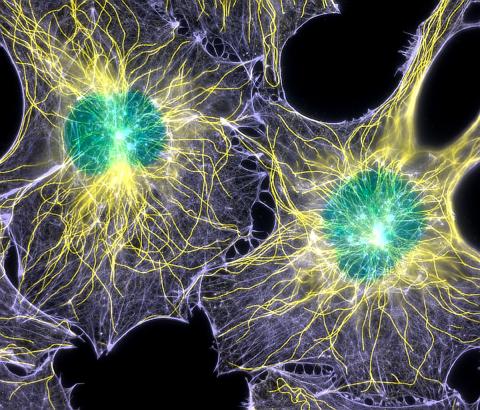
2428: Colorful cells
2428: Colorful cells
Actin (purple), microtubules (yellow), and nuclei (green) are labeled in these cells by immunofluorescence. This image won first place in the Nikon 2003 Small World photo competition.
Torsten Wittmann, Scripps Research Institute
View Media
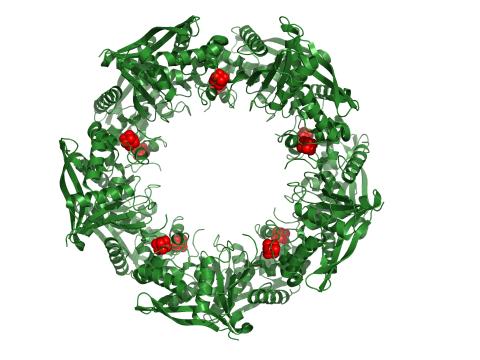
3720: Cas4 nuclease protein structure
3720: Cas4 nuclease protein structure
This wreath represents the molecular structure of a protein, Cas4, which is part of a system, known as CRISPR, that bacteria use to protect themselves against viral invaders. The green ribbons show the protein's structure, and the red balls show the location of iron and sulfur molecules important for the protein's function. Scientists harnessed Cas9, a different protein in the bacterial CRISPR system, to create a gene-editing tool known as CRISPR-Cas9. Using this tool, researchers are able to study a range of cellular processes and human diseases more easily, cheaply and precisely. In December, 2015, Science magazine recognized the CRISPR-Cas9 gene-editing tool as the "breakthrough of the year." Read more about Cas4 in the December 2015 Biomedical Beat post A Holiday-Themed Image Collection.
Fred Dyda, NIDDK
View Media
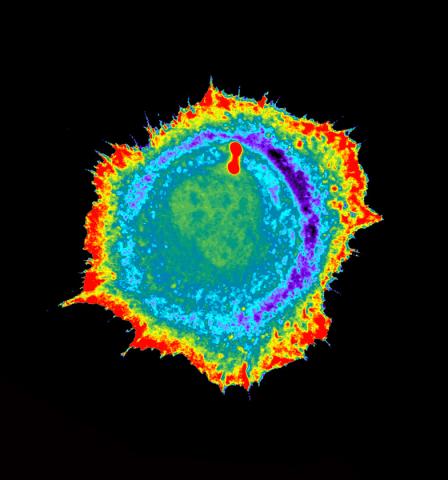
2451: Seeing signaling protein activation in cells 01
2451: Seeing signaling protein activation in cells 01
Cdc42, a member of the Rho family of small guanosine triphosphatase (GTPase) proteins, regulates multiple cell functions, including motility, proliferation, apoptosis, and cell morphology. In order to fulfill these diverse roles, the timing and location of Cdc42 activation must be tightly controlled. Klaus Hahn and his research group use special dyes designed to report protein conformational changes and interactions, here in living neutrophil cells. Warmer colors in this image indicate higher levels of activation. Cdc42 looks to be activated at cell protrusions.
Related to images 2452, 2453, and 2454.
Related to images 2452, 2453, and 2454.
Klaus Hahn, University of North Carolina, Chapel Hill Medical School
View Media
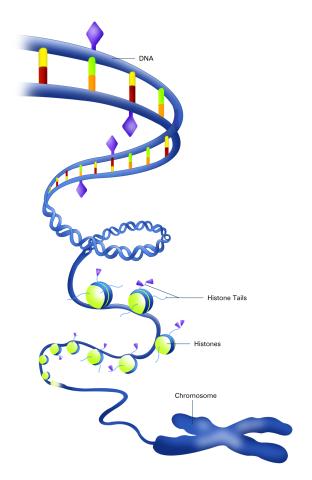
2563: Epigenetic code (with labels)
2563: Epigenetic code (with labels)
The "epigenetic code" controls gene activity with chemical tags that mark DNA (purple diamonds) and the "tails" of histone proteins (purple triangles). These markings help determine whether genes will be transcribed by RNA polymerase. Genes hidden from access to RNA polymerase are not expressed. See image 2562 for an unlabeled version of this illustration. Featured in The New Genetics.
Crabtree + Company
View Media
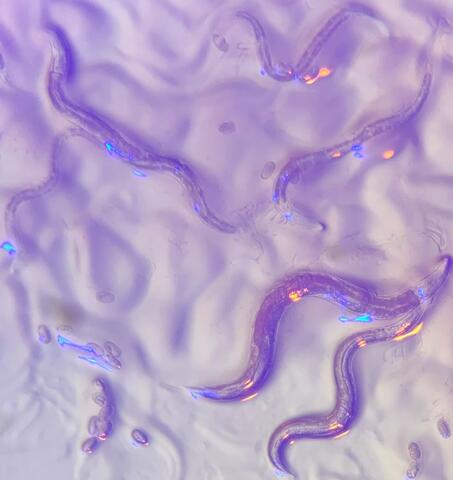
6750: C. elegans with blue and yellow lights in the background
6750: C. elegans with blue and yellow lights in the background
These microscopic roundworms, called Caenorhabditis elegans, lack eyes and the opsin proteins used by visual systems to detect colors. However, researchers found that the worms can still sense the color of light in a way that enables them to avoid pigmented toxins made by bacteria. This image was captured using a stereo microscope.
H. Robert Horvitz and Dipon Ghosh, Massachusetts Institute of Technology.
View Media
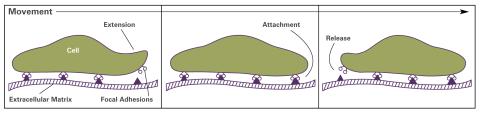
2503: Focal adhesions (with labels)
2503: Focal adhesions (with labels)
Cells walk along body surfaces via tiny "feet," called focal adhesions, that connect with the extracellular matrix. See image 2502 for an unlabeled version of this illustration.
Crabtree + Company
View Media
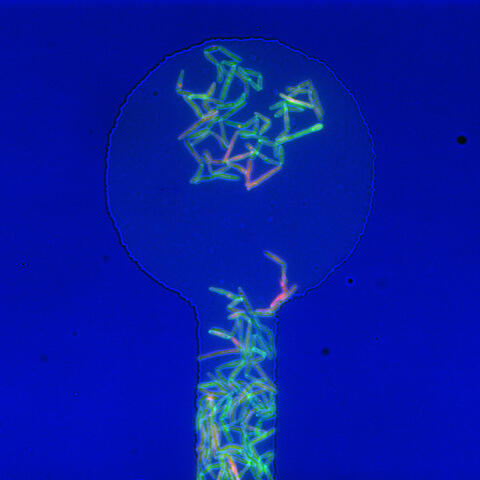
5751: Genetically identical mycobacteria respond differently to antibiotic 1
5751: Genetically identical mycobacteria respond differently to antibiotic 1
Antibiotic resistance in microbes is a serious health concern. So researchers have turned their attention to how bacteria undo the action of some antibiotics. Here, scientists set out to find the conditions that help individual bacterial cells survive in the presence of the antibiotic rifampicin. The research team used Mycobacterium smegmatis, a more harmless relative of Mycobacterium tuberculosis, which infects the lung and other organs and causes serious disease.
In this image, genetically identical mycobacteria are growing in a miniature growth chamber called a microfluidic chamber. Using live imaging, the researchers found that individual mycobacteria will respond differently to the antibiotic, depending on the growth stage and other timing factors. The researchers used genetic tagging with green fluorescent protein to distinguish cells that can resist rifampicin and those that cannot. With this gene tag, cells tolerant of the antibiotic light up in green and those that are susceptible in violet, enabling the team to monitor the cells' responses in real time.
To learn more about how the researchers studied antibiotic resistance in mycobacteria, see this news release from Tufts University. Related to video 5752.
In this image, genetically identical mycobacteria are growing in a miniature growth chamber called a microfluidic chamber. Using live imaging, the researchers found that individual mycobacteria will respond differently to the antibiotic, depending on the growth stage and other timing factors. The researchers used genetic tagging with green fluorescent protein to distinguish cells that can resist rifampicin and those that cannot. With this gene tag, cells tolerant of the antibiotic light up in green and those that are susceptible in violet, enabling the team to monitor the cells' responses in real time.
To learn more about how the researchers studied antibiotic resistance in mycobacteria, see this news release from Tufts University. Related to video 5752.
Bree Aldridge, Tufts University
View Media
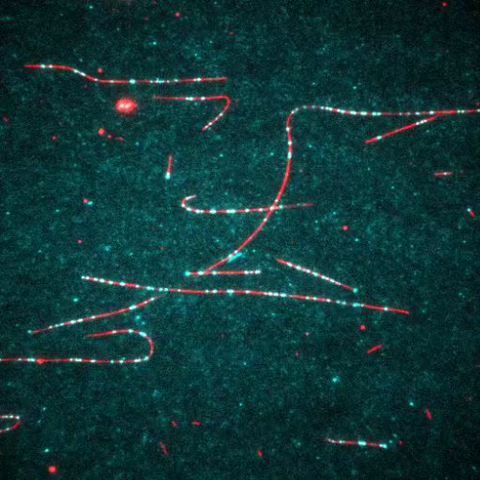
7023: Dynein moving along microtubules
7023: Dynein moving along microtubules
Dynein (green) is a motor protein that “walks” along microtubules (red, part of the cytoskeleton) and carries its cargo along with it. This video was captured through fluorescence microscopy.
Morgan DeSantis, University of Michigan.
View Media
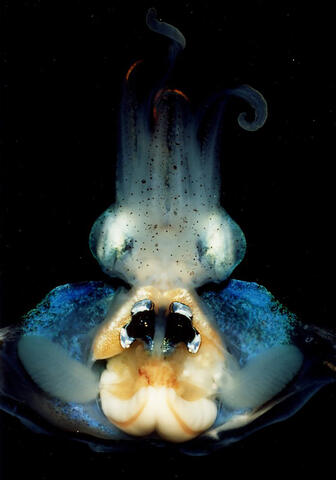
7013: An adult Hawaiian bobtail squid
7013: An adult Hawaiian bobtail squid
An adult female Hawaiian bobtail squid, Euprymna scolopes, with its mantle cavity exposed from the underside. Some internal organs are visible, including the two lobes of the light organ that contains bioluminescent bacteria, Vibrio fischeri. The light organ includes accessory tissues like an ink sac (black) that serves as a shutter, and a silvery reflector that directs the light out of the underside of the animal.
Margaret J. McFall-Ngai, Carnegie Institution for Science/California Institute of Technology, and Edward G. Ruby, California Institute of Technology.
View Media
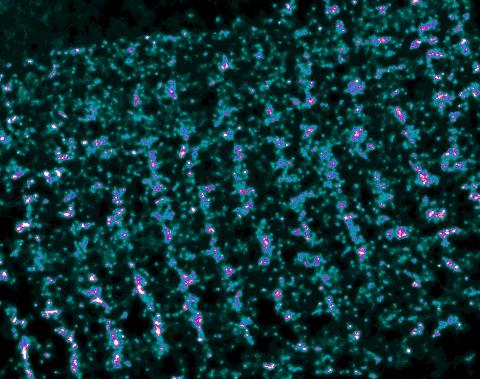
3339: Single-Molecule Imaging
3339: Single-Molecule Imaging
This is a super-resolution light microscope image taken by Hiro Hakozaki and Masa Hoshijima of NCMIR. The image contains highlighted calcium channels in cardiac muscle using a technique called dSTORM. The microscope used in the NCMIR lab was built by Hiro Hakozaki.
Tom Deerinck, NCMIR
View Media

2762: Nucleolinus
2762: Nucleolinus
The nucleolinus is a cellular compartment that has been a lonely bystander in scientific endeavors. Although it's found in a range of species, its function has been mysterious—mainly because the structure is hard to visualize. An August 2010 study showed that the nucleolinus is crucial for cell division. When researchers zapped the structure with a laser, an egg cell didn't complete division. When the oocyte was fertilized after laser microsurgery (bottom right), the resulting zygote didn't form vital cell division structures (blue and yellow).
Mary Anne Alliegro, Marine Biological Laboratory
View Media
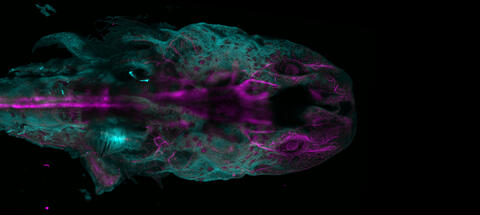
6927: Axolotl showing nervous system
6927: Axolotl showing nervous system
The head of an axolotl—a type of salamander—that has been genetically modified so that its developing nervous system glows purple and its Schwann cell nuclei appear light blue. Schwann cells insulate and provide nutrients to peripheral nerve cells. Researchers often study axolotls for their extensive regenerative abilities. They can regrow tails, limbs, spinal cords, brains, and more. The researcher who took this image focuses on the role of the peripheral nervous system during limb regeneration.
This image was captured using a light sheet microscope.
Related to images 6928 and 6932.
This image was captured using a light sheet microscope.
Related to images 6928 and 6932.
Prayag Murawala, MDI Biological Laboratory and Hannover Medical School.
View Media
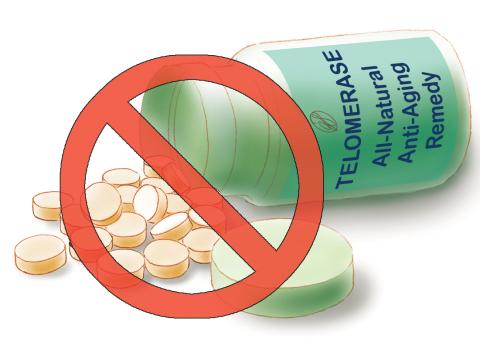
1335: Telomerase illustration
1335: Telomerase illustration
Reactivating telomerase in our cells does not appear to be a good way to extend the human lifespan. Cancer cells reactivate telomerase.
Judith Stoffer
View Media
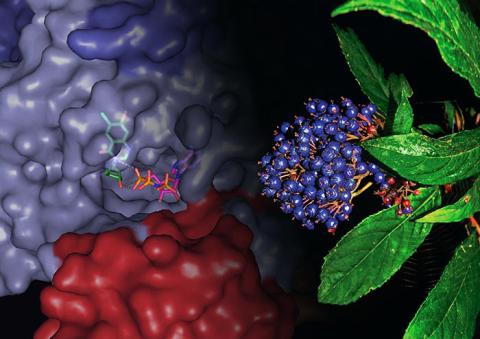
3483: Chang Shan
3483: Chang Shan
For thousands of years, Chinese herbalists have treated malaria using Chang Shan, a root extract from a type of hydrangea that grows in Tibet and Nepal. Recent studies have suggested Chang Shan can also reduce scar formation, treat multiple sclerosis and even slow cancer progression.
Paul Schimmel Lab, Scripps Research Institute
View Media
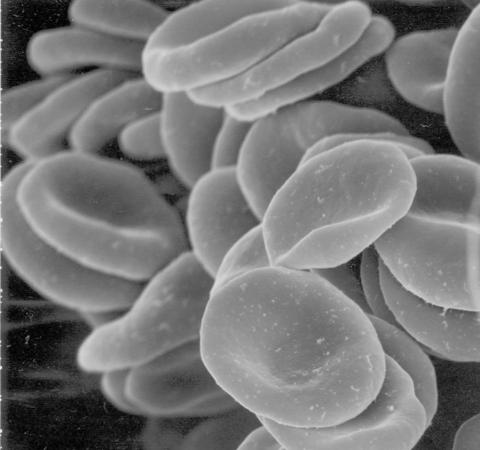
1101: Red blood cells
1101: Red blood cells
This image of human red blood cells was obtained with the help of a scanning electron microscope, an instrument that uses a finely focused electron beam to yield detailed images of the surface of a sample.
Tina Weatherby Carvalho, University of Hawaii at Manoa
View Media
2795: Anti-tumor drug ecteinascidin 743 (ET-743), structure without hydrogens 02
2795: Anti-tumor drug ecteinascidin 743 (ET-743), structure without hydrogens 02
Ecteinascidin 743 (ET-743, brand name Yondelis), was discovered and isolated from a sea squirt, Ecteinascidia turbinata, by NIGMS grantee Kenneth Rinehart at the University of Illinois. It was synthesized by NIGMS grantees E.J. Corey and later by Samuel Danishefsky. Multiple versions of this structure are available as entries 2790-2797.
Timothy Jamison, Massachusetts Institute of Technology
View Media
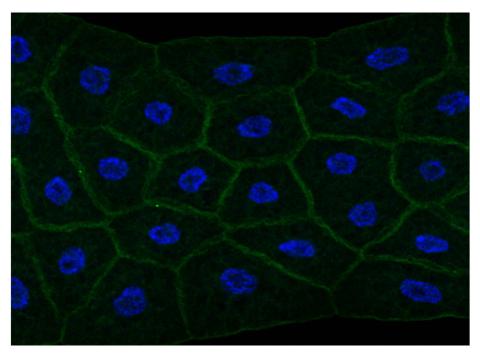
2757: Draper, shown in the fatbody of a Drosophila melanogaster larva
2757: Draper, shown in the fatbody of a Drosophila melanogaster larva
The fly fatbody is a nutrient storage and mobilization organ akin to the mammalian liver. The engulfment receptor Draper (green) is located at the cell surface of fatbody cells. The cell nuclei are shown in blue.
Christina McPhee and Eric Baehrecke, University of Massachusetts Medical School
View Media
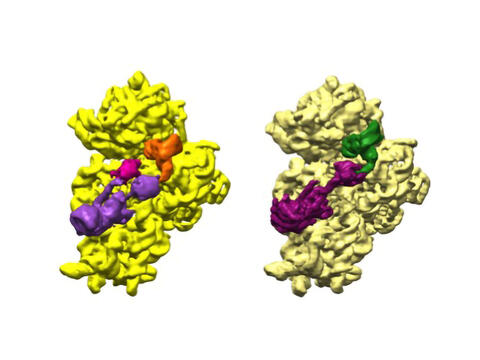
6578: Bacterial ribosome assembly
6578: Bacterial ribosome assembly
3D reconstructions of two stages in the assembly of the bacterial ribosome created from time-resolved cryo-electron microscopy images. Ribosomes translate genetic instructions into proteins.
Joachim Frank, Columbia University.
View Media
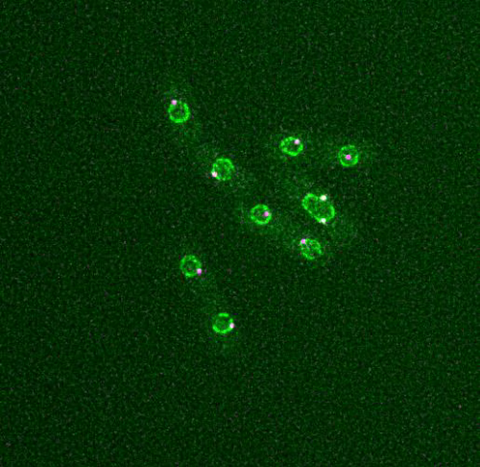
6795: Dividing yeast cells with nuclear envelopes and spindle pole bodies
6795: Dividing yeast cells with nuclear envelopes and spindle pole bodies
Time-lapse video of yeast cells undergoing cell division. Nuclear envelopes are shown in green, and spindle pole bodies, which help pull apart copied genetic information, are shown in magenta. This video was captured using wide-field microscopy with deconvolution.
Related to images 6791, 6792, 6793, 6794, 6797, 6798, and video 6796.
Related to images 6791, 6792, 6793, 6794, 6797, 6798, and video 6796.
Alaina Willet, Kathy Gould’s lab, Vanderbilt University.
View Media
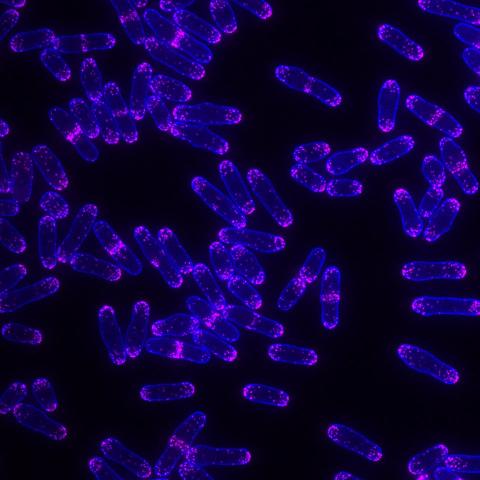
6794: Yeast cells with Fimbrin Fim1
6794: Yeast cells with Fimbrin Fim1
Yeast cells with the protein Fimbrin Fim1 shown in magenta. This protein plays a role in cell division. This image was captured using wide-field microscopy with deconvolution.
Related to images 6791, 6792, 6793, 6797, 6798, and videos 6795 and 6796.
Related to images 6791, 6792, 6793, 6797, 6798, and videos 6795 and 6796.
Alaina Willet, Kathy Gould’s lab, Vanderbilt University.
View Media
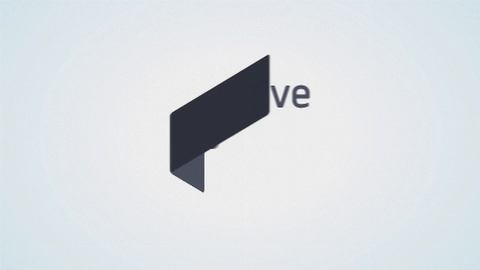
5815: Introduction to Genome Editing Using CRISPR/Cas9
5815: Introduction to Genome Editing Using CRISPR/Cas9
Genome editing using CRISPR/Cas9 is a rapidly expanding field of scientific research with emerging applications in disease treatment, medical therapeutics and bioenergy, just to name a few. This technology is now being used in laboratories all over the world to enhance our understanding of how living biological systems work, how to improve treatments for genetic diseases and how to develop energy solutions for a better future.
Janet Iwasa
View Media
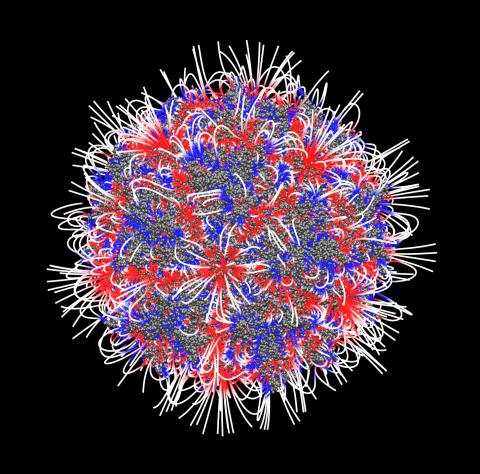
3374: Electrostatic map of the adeno-associated virus
3374: Electrostatic map of the adeno-associated virus
The new highly efficient parallelized DelPhi software was used to calculate the potential map distribution of an entire virus, the adeno-associated virus, which is made up of more than 484,000 atoms. Despite the relatively large dimension of this biological system, resulting in 815x815x815 mesh points, the parallelized DelPhi, utilizing 100 CPUs, completed the calculations within less than three minutes. Related to image 3375.
Emil Alexov, Clemson University
View Media
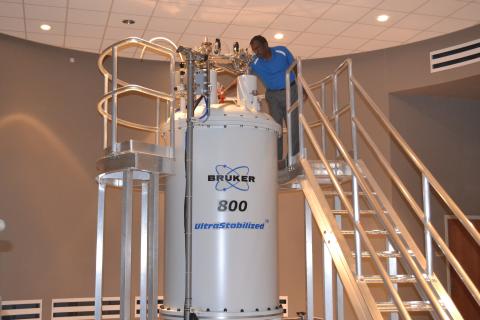
3526: 800 MHz NMR magnet
3526: 800 MHz NMR magnet
Scientists use nuclear magnetic spectroscopy (NMR) to determine the detailed, 3D structures of molecules.
Asokan Anbanandam, University of Kansas
View Media
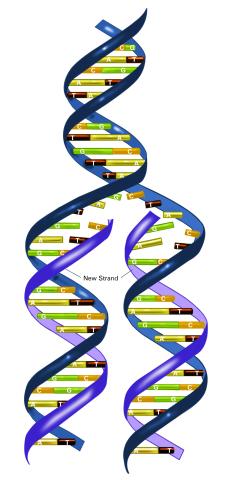
2544: DNA replication illustration (with labels)
2544: DNA replication illustration (with labels)
During DNA replication, each strand of the original molecule acts as a template for the synthesis of a new, complementary DNA strand. See image 2543 for an unlabeled version of this illustration. Featured in The New Genetics.
Crabtree + Company
View Media
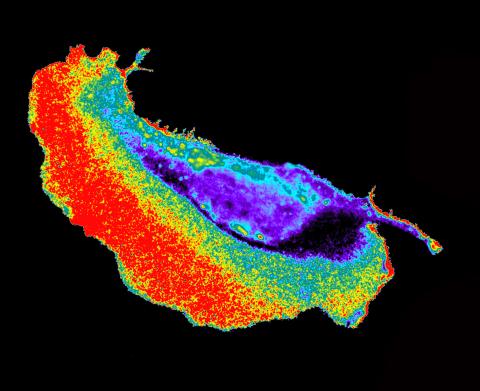
2452: Seeing signaling protein activation in cells 02
2452: Seeing signaling protein activation in cells 02
Cdc42, a member of the Rho family of small guanosine triphosphatase (GTPase) proteins, regulates multiple cell functions, including motility, proliferation, apoptosis, and cell morphology. In order to fulfill these diverse roles, the timing and location of Cdc42 activation must be tightly controlled. Klaus Hahn and his research group use special dyes designed to report protein conformational changes and interactions, here in living neutrophil cells. Warmer colors in this image indicate higher levels of activation. Cdc42 looks to be activated at cell protrusions.
Related to images 2451, 2453, and 2454.
Related to images 2451, 2453, and 2454.
Klaus Hahn, University of North Carolina, Chapel Hill Medical School
View Media